Updated Projections of Canada’s Public Charging Infrastructure Needs
Download
full document
(PDF, 2.1 MB)
UPDATED PROJECTIONS
PREPARED FOR: Natural Resources Canada
March 31, 2022
Submitted to:
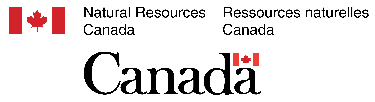
Natural Resources Canada
Thierry Spiess
Senior Manager
Advanced Vehicle Programming
613-617-1458 | thierry.spiess@nrcan-rncan.gc.ca
www.nrcan.gc.ca
Prepared by:
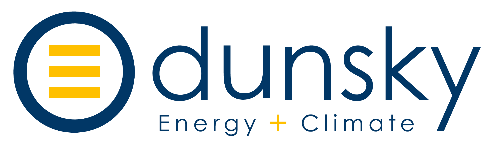
Dunsky Energy + Climate Advisors
50 Ste-Catherine St. West, Suite 420
Montreal, QC H2X 3V4
www.dunsky.com | info@dunsky.com
+ 1-514-504-9030
About Dunsky
18
years
40+
dedicated
Professionals
600+
Projects across
30 states and Provineces.
Founded in 2004, Dunsky supports leading governments, utilities, corporations and non-profit organizations across North America in their efforts to accelerate the clean energy transition, effectively and responsibly.
Working across buildings, industry, energy and mobility, we support our clients through three key services: we quantify opportunities (technical, economic, market), design go-to-market strategies (plans, programs, policies) and evaluate performance (with a view to continuous improvement).
Dunsky is proudly Canadian, with offices and staff in Montreal, Toronto, Vancouver, Ottawa and Halifax.
EXECUTIVE SUMMARY
Context
As part of its commitment to achieving net zero greenhouse gas (GHG) emissions by 2050, the Government of Canada recently adopted a mandatory target of achieving 100% zero-emission vehicle market share for new light-duty vehicle (LDV) sales by 2035. Achieving this target will require collaboration across a wide range of actors, including the federal government, municipal and provincial governments, electric utilities, the automotive industry and other private organizations. In recent years, the federal government has made significant contributions to accelerate the adoption of zero-emission vehicles (ZEVs), including public awareness and education campaigns, purchase rebates, and investments in electric vehicle (EV) charging infrastructure.
Through a range of initiatives, Natural Resources Canada has supported the deployment of thousands of EV charging stations across Canada since 2016. While natural market forces are likely to help guide charging infrastructure requirements in the long term, challenging business models around charging infrastructure mean that ongoing collaboration between governments, utilities and the private sector will be required for the foreseeable future.
In order to inform future program development and maximize collaboration, this study was commissioned to improve understanding of Canada’s overall charging infrastructure needs and how these are likely to evolve as the national EV fleet continues to grow. This study builds on a previous analysis that Dunsky conducted in 2018, updating this analysis to reflect an accelerated timeline for achieving 100% ZEV market share of new sales.
Charging Needs Are Driven by a Growing EV Population
This study makes use of the concept of EV-to-charger ratios. Expressing charging needs in terms of a ratio helps to understand how charging infrastructure must grow to meet the needs of a growing EV population over time.
Transport Canada and NRCan provided Dunsky with scenarios for EV adoption based on the federal target of achieving 100% market share of new LDV sales by 2035. While interim targets had not yet been finalized at the time of the study, we used a scenario developed by Transport Canada that achieves a 15% market share of new vehicle sales by 2025 and a 60% market share by 2030.
2020 | 2025 | 2030 | 2035 | 2040 | 2045 | 2050 | |
---|---|---|---|---|---|---|---|
EVs in circulation | 203,150 | 1,019,009 | 4,632,759 | 12,366,822 | 20,651,792 | 26,933,160 | 31,010,664 |
% of LDV Fleet | 0.8% | 3.8% | 16% | 40% | 63% | 80% | 90% |
Different Charging Types Serve Different Purposes
While most EV drivers are expected to do most of their charging at home, this study focuses on assessing needs for public charging infrastructure. There are two primary types of public charging infrastructure:
- Level 2 (“L2”) charging can typically recharge a Battery Electric Vehicle (BEV) in roughly eight (8) hours or a Plug-in Hybrid Vehicle (PHEV) in roughly four (4) hours. L2 charging is useful for charging in locations where vehicles remain parked for several hours, either during the day or overnight.
- DC Fast Charging (“DCFC”) can typically recharge an EV to 80% charge in roughly 30 minutes, depending on the charger output power and the EV’s battery size. DCFCs are typically only used by BEVs.
Our analysis breaks down the needs for charging infrastructure into two overall use cases:
- Highway corridor charging is critical to enable BEVs to travel long distances, relying exclusively on DCFC infrastructure. Corridor charging must provide adequate geographic coverage or connectivity to ensure that BEV drivers can get where they need to go. In order to avoid lineups, corridor charging must also have sufficient charging capacity to charge the expected volume of BEVs, both in terms of the number of charging ports and the charging speed available.
- Community cluster charging, including both L2 and DCFC infrastructure, supports both PHEV and BEV drivers by providing charging access within population centres. This can include public charging hubs at retail locations, curbside charging, and workplace charging. The needs for public charging within cities is closely linked to the degree to which EV owners have access to charging at home, given that public charging can serve as a substitute for EV owners who cannot charge at home.
Our analysis accounts for a wide range of factors that can impact the overall charging needs, including cold climate impacts, EV energy consumption ratings and charging speeds, and the level of home charging access over time.
Results
The table below outlines the total public charging infrastructure needs and EV-to-charger ratios for Canada, combining both highway corridor and community cluster infrastructure, and including assumptions related to home charging access. We include two scenarios representing different levels of progress in improving home charging access in multi-unit residential buildings (MURBs), highlighting the reduction in demand for public charging infrastructure if more EV owners can charge at home.
2025 | 2030 | 2035 | 2040 | 2045 | 2050 | ||
---|---|---|---|---|---|---|---|
Scenario 1: High access to home charging |
Public DCFC | 4,300 | 13,800 | 32,000 | 50,200 | 62,700 | 69,000 |
Public L2 | 48,000 | 181,000 | 410,000 | 593,000 | 673,000 | 658,000 | |
Total Public Ports | 52,000 | 195,000 | 442,000 | 643,000 | 736,000 | 727,000 | |
Total MURB Ports | 515,000 | 1,302,000 | 2,189,000 | 3,191,000 | 4,326,000 | 5,610,000 | |
EVs/Level 2 | 21 | 26 | 30 | 35 | 40 | 47 | |
BEVs/DCFC | 180 | 250 | 300 | 330 | 350 | 380 | |
EVs/Public Port | 20 | 24 | 28 | 32 | 37 | 43 | |
EVs/Total Ports | 2 | 3 | 5 | 5 | 5 | 5 | |
Scenario 2: Low access to home charging |
Total DCFC | 4,300 | 14,100 | 33,700 | 55,100 | 72,500 | 84,900 |
Total L2 | 49,000 | 186,000 | 436,000 | 659,000 | 791,000 | 830,000 | |
Total Ports | 53,000 | 201,000 | 469,000 | 714,000 | 864,000 | 914,000 | |
Total MURB Ports | 46,000 | 152,000 | 499,000 | 886,000 | 1,318,000 | 1,799,000 | |
EVs/Level 2 | 21 | 25 | 28 | 31 | 34 | 37 | |
BEVs/DCFC | 170 | 240 | 280 | 300 | 300 | 310 | |
EVs/Public Port | 20 | 23 | 26 | 29 | 31 | 34 | |
EVs/Total Ports | 11 | 14 | 13 | 13 | 13 | 12 |
The findings of our updated analysis highlight the need for significant ongoing investment in public charging infrastructure across Canada. While the results are largely consistent with our previous analysis, we note a few key findings below:
- Compared to our 2018 study, we see a need for a significant acceleration in charging infrastructure deployment over the next five to ten years in order to support the federal government’s target of achieving 100% EV market share of new light-duty vehicle sales by 2035. By 2025, we see a need for 4,300 DCFC ports across Canada, a significant increase from the 3,800 ports that we predicted in our 2018 study and from the roughly 3,000 DCFC ports currently installed as of November 2021.
- In the very long term, our overall estimate for public charging infrastructure needs across Canada would represent a total investment of approximately $20 billion over the next three decades. However, while this analysis quantifies the total infrastructure needed to meet Canada’s EV adoption targets, this study does not assess what portion of this infrastructure would require support from the federal government. The federal government is currently leveraging significant co-investment from other actors in the EV charging ecosystem, and we can expect the overall proportion of federal investment in charging infrastructure to decline as this ecosystem becomes increasingly competitive and attracts investment from other actors.
- As we saw in our 2018 study, the primary driver for the number of charging ports is capacity requirements within community clusters. While infrastructure along highway corridors is essential to ensure connectivity, the long distances between populated areas in Canada mean that many of these corridors do not serve high volumes. Charging within population centres represents the bulk of the needs for charging infrastructure deployment.
- The results from our two scenarios for different levels of home charging access highlight the benefits of taking aggressive actions to improve home charging access and reducing overall demand on public charging infrastructure. Charging at home overnight is the most convenient option for EV owners and can also be the most cost-effective option when charging infrastructure is deployed at scale and incorporated into new buildings during construction. Ongoing efforts by the federal government to retrofit existing buildings and ensure new buildings are designed with EV charging in mind will lead to significant cost savings through reduced needs for public charging, while also making EV ownership more convenient for a broader range of Canadian households.
- For households that do not have access to charging at home, public charging infrastructure can potentially serve as a substitute. While this is typically less convenient and more expensive than charging at home, it may be the only option for some households. The relative importance of DCFC and L2 infrastructure as a home-charging substitute will depend on a number of factors, including cost, convenience, and cold weather impacts. Finding the right mix of investments in charging infrastructure in community clusters will benefit from strong participation from local governments.
Overall, the results presented in this study represent our best estimate of what will be needed to support Canada’s ZEV adoption targets over the coming decades. There is significant uncertainty in a number of aspects of the analysis as discussed above, and unforeseen technology advancements could alter Canada’s ZEV infrastructure pathway significantly. Ultimately, investments in charging infrastructure can grow over time in response to anticipated demand on a shorter timescale than the decades-long outlook presented here. But laying out a roadmap for future infrastructure needs based on our best understanding today is essential to guide near-term policies and investments, and identify gaps in our understanding that warrant further analysis.
Table of Contents
1.0 Introduction
1.1 Context
The Government of Canada has committed to achieving net zero greenhouse gas (GHG) emissions by 2050. With the transportation sector representing a major portion of Canada’s GHGs, the transition towards Zero-Emission Vehicles (ZEVs) is a crucial component of Canada’s net zero commitment. As such, the federal government recently adopted a mandatory target of achieving 100% ZEV market share for new light-duty vehicle (LDV) sales by 2035.
Achieving Canada’s ZEV adoption targets will require collaboration across a wide range of actors, including the federal government, municipal and provincial governments, electric utilities, the automotive industry, and other private organizations. In recent years, the federal government has made significant contributions to accelerate the adoption of ZEVs, including public awareness and education campaigns, purchase rebates, and investments in electric vehicle (EV) charging infrastructure. Through a range of initiatives, Natural Resources Canada has supported the deployment of thousands of EV charging stations across Canada since 2012.
While natural market forces are likely to help guide charging infrastructure requirements in the long term, challenging business models around charging infrastructure mean that ongoing collaboration between governments, utilities and the private sector will be required for the foreseeable future. In order to inform future program development and to maximize collaboration, it is important to understand the overall charging infrastructure needs and how these are likely to evolve as the national EV fleet continues to grow.
1.2 Background of This Study
In 2018, Dunsky was retained to support the development of an appropriate ratio of EVs to public chargers that could guide future deployments and maximize their impact on the adoption of EVs. The report was intended to provide high-level guidance on charging infrastructure requirements at the national scale based on the previous federal target of achieving 100% ZEV market share of new LDVs by 2040.
Since then, the federal government has brought this target forward by five (5) years (100% by 2035), has introduced the Incentives for Zero-Emission Vehicles Program (iZEV) offering purchase rebates, has funded a range of EV awareness initiatives, and has continued to provide significant funding for charging infrastructure deployment through the Electric Vehicle and Alternative Fuel Infrastructure Deployment Initiative (EVAFIDI) and the Zero-Emission Vehicle Infrastructure Program (ZEVIP). Meanwhile, the EV market continues to evolve at a rapid pace, with a diverse range of EV models available across a growing number of vehicle segments, declining EV purchase prices, and increasing EV capabilities in terms of driving range and fast charging capability.
Given the ongoing evolution of the EV market, NRCan asked Dunsky to perform a review of our previous study and to identify opportunities for updating our methodology and revising our estimates for optimal EV-to-charger ratios and total infrastructure needs, in order to support Canada’s EV adoption targets.
This report is intended to serve as an update to our previous report and approach, while providing a standalone reference that describes our entire methodology. As such, the description of our methodology includes content that was carried over from the previous report, with updates noted where relevant.
1.3 Definitions
To ensure consistency, the following definitions will be used throughout the report:
- Electric Vehicle (EV): An EV is a vehicle that uses one or more electric motors for propulsion with onboard energy storage that is recharged by plugging it into an external source of electric power. For the purposes of this report, all EVs are light-duty vehicles (i.e., with a weight rating of 4,500 kg or less).
- Battery Electric Vehicle (BEV): A BEV is a type of EV that only uses electric motors and the energy stored in its rechargeable battery packs. It does not use an internal combustion engine (ICE).
- Plug-in Hybrid Electric Vehicle (PHEV): A PHEV is a type of EV that includes both electric motors and an ICE. Its battery can be recharged by plugging it into an external source of electric power.
- Public Chargers: These encompass all chargers not located at residential locations. Although some workplace charging is restricted to certain users, we include workplace charging in the public charging category.
- Charging Site: An EV charging site is an infrastructure location where electric energy can be supplied to recharge EVs. A charging site can have multiple charging ports. (Note that our previous report used the term “Charging Station,” but we have shifted to “Charging Site” to avoid confusion, given that “Charging Station” is often used to refer to a single piece of charging equipment.)
- Charging Port:Footnote 1 An EV charging port is the outlet used to charge the EV. The port can be located at a charging site. Although not always technically correct, the term “charger” is often used to refer to a charging port for simplicity’s sake.
- DC Fast Charger (DCFC): A type of charging port that allows for faster charging of the EV. With current technology, drivers can typically charge 80% of their battery in 30 minutes.
- Level 2 (L2) Charger: A type of charging port that can provide power at 240 volts and up to 30 amps. Drivers can typically add 15 to 40 km of range in one hour of charging.
1.4 2018 Dunsky Charging Infrastructure Needs Projections
In our 2018 study, we developed optimal ratios of “EVs-to-chargers” for both DCFC and L2 charging infrastructure and estimated total charging infrastructure needs through to 2050. Our overall approach to developing these estimates included:
- conducting a literature review of previous research and forecasts of charging infrastructure needs;
- engaging with stakeholders involved in EV infrastructure deployment in a range of jurisdictions, including Quebec, B.C., France and Norway;
- developing a methodology for estimating charging infrastructure needs adapted to the Canadian context.
This methodology included estimates for DCFC infrastructure required along highway corridors, and both DCFC and L2 infrastructure required in population centres. The overall results from the 2018 study are presented in the table below:
2020 | 2025 | 2030 | 2035 | 2040 | 2045 | 2050 | |
---|---|---|---|---|---|---|---|
Total DCFC | 1,000 | 3,800 | 11,600 | 33,000 | 50,800 | 63,700 | 77,600 |
Total L2 | 15,000 | 42,000 | 101,000 | 240,000 | 361,000 | 443,000 | 536,000 |
Total Ports | 16,000 | 45,000 | 112,000 | 273,000 | 412,000 | 506,000 | 614,000 |
EVs/Level 2 | 15 | 22 | 31 | 41 | 46 | 53 | 56 |
BEVs/DCFC | 140 | 180 | 220 | 260 | 290 | 330 | 350 |
EVs/Ports | 14 | 20 | 27 | 36 | 41 | 46 | 49 |
In the following section, we provide an overview of our approach for updating this analysis.
2.0 Updating Our Approach
Given the ongoing evolution of the EV market, this study provides an opportunity to review the latest research and re-evaluate the methodology employed in our 2018 study. This section provides an overview of our findings and the updates that we identified for use in this study.
2.1 Scan of Emerging Methodologies and Results (2019‒2021)
While a full jurisdictional scan was not conducted for the updated analysis, a desktop review of recent research was undertaken to help assess the validity of Dunsky’s methodology and to review the latest research. Several reports were reviewed, including:
- ICCT (International Council on Clean Transportation) - Charging up America report, July 2021 Footnote 2
- Atlas Public Policy - U.S. Passenger Vehicle Electrification Infrastructure Assessment, April 2021 Footnote 3
- The Brattle Group - Getting to 20 Million EVs by 2030, June 2020 Footnote 4
- McKinsey Center for Future Mobility - Charging Ahead: Electric Vehicle Infrastructure Demand, October 2018 Footnote 5
- M.J. Bradley & Associates (MJB&A) - Regional EV Charging Infrastructure Location Identification Toolkit, September 2021 Footnote 6
- National Renewable Energy Laboratory (NREL) Analysis - National Plug-In Electric Vehicle Infrastructure Analysis, 2017 Footnote 7
We found that much of the research still relies heavily on the 2017 National Renewable Energy Laboratory (NREL) analysis, the same analysis that we used as a starting point for nominal EV-to-charger ratios for population clusters in our 2018 study.
We also found that our approach to have separate modeling for corridors and for population clusters is still common practice. While our methodology was found to be in line with current research methods, results differed based on varying assumptions and factors.
The ICCT published a summary table of previous EV infrastructure requirements studies, shown in Table 2, highlighting the variety of research due to varying assumptions and factors. The paper found an average of 37 EVs per public charger.
Study | Projection year | Scale | EV sales (%) | EV fleet size | EVs per public charger |
---|---|---|---|---|---|
Wood Mackenzie (2019) | 2025 | North America | N/A | 15.4 M | 29.8 |
Crisostomo et al. (2021) | 2030 | California | N/A | 5 M | 12 |
Cooper & Schefter (2018) | 2030 | United States | 22 | 18.7 M | 20.8 |
Engel et al. (2018) | 2030 | United States | 14 | 18 M | 27.7 |
Stock (2020) | 2040 | Europe and United States | 58 | NA | 45 |
McKenzie et al. (2021) | 2035 | United States | 100 | 115 M | 129 |
ICCT | 2030 | United States | 36 | 26 M | 24 |
As was noted in our 2018 study, it is important to note that an apples-to-apples comparison of ratios between jurisdictions is difficult due to differences between:
- Reporting Methods: with no standardized method to report on EV or charger counts, definitions can differ between jurisdictions, resulting in ratios that are not comparable; and
- Impact Factors: differences between jurisdictions limit the applicability of a given ratio from one jurisdiction to another.
These factors are described in more detail below.
2.1.1 Reporting methods may vary
There are several factors that can impact the counts being reported by jurisdictions:
- Charging Infrastructure Varies: When calculating the ratio of EVs to charger, jurisdictions often use different definitions of chargers. This is due to the fact that several types of charging infrastructure are available (see Table 3).
Table 3: Three levels of charging infrastructure currently exist Level 1 Level 2 DCFC (“Level 3”) Typical output 120 Volts/12 Amps 240 Volts/30 Amps 50‒350 kW Charging time (to replenish 120 km of range) 16+ hours 4 hours 5–25 minutes Installation costs $200‒$1,500 $2,000‒$10,000 $50,000‒$100,000+ Typical locations Homes, offices Homes, offices, public spaces Corridors, public spaces Used by BEVs and PHEVs BEVs and PHEVs Primarily BEVsFootnote 8 - Public Charging Definition Varies: When looking to identify the ratio of EVs to public charger, it can be difficult to understand how jurisdictions are defining “public charger.” For example, in some cases workplace charging is included, while in others it is not. Our analysis includes workplace charging as a subset of public charging, given that it can be challenging to draw a line between charging infrastructure in dedicated employee parking spots vs charging infrastructure in public parking lots used by employees.
- Electric Vehicle Type Varies: With respect to the EV portion of the ratio, jurisdictions tend to report their ratios using total EVs (a combination of BEVs and PHEVs), or based solely on BEVs. Typically, ratios related to DCFC infrastructure is based on BEV counts. This is also inconsistent between jurisdictions.
2.1.2 Impact Factors Change Regional Needs
Even if reporting is done using the same definition for EVs and chargers, the potential remains for a large difference in infrastructure needs between jurisdictions due to several factors:
- Geography: A higher population density can increase the allowable ratio of EVs to chargers by allowing for more effective use of the charging infrastructure. As such, large urban centres typically allow a higher ratio of EVs to chargers (i.e., fewer chargers for a given population of EVs) than do rural communities.
- In addition, difference in terrain can increase energy needs. For example, the mountainous terrain in British Columbia could result in a greater need for chargers than that required in the relatively flat Prairies.
- Climate: As temperatures move towards the cold or hot extremes, there are negative impacts on vehicle and battery efficiency, resulting in greater charging needs.
- EV Type: BEVs have a greater need for DCFC infrastructure, whereas PHEVs have alternative fuel sources that they can use when requiring additional range. Level 2 charging infrastructure tends to support all EV types. It is important to understand the split between BEVs and PHEVs, and the associated driving ranges for each type of EV.
- Battery Capacity: As technology improves, battery capacity is also expected to increase. However, it is likely that the industry will reach an optimal maximum battery capacity, at which point the focus will shift to optimizing other factors (e.g., affordability, comfort).
- Charging Speed: Charging speeds are also expected to improve over time. While 50 kW was still the norm in 2018, many EVs coming to market today and in the near future support charging at over 200 kW, even as high as 350 kW. This may reduce typical DCFC charging times from approximately 30 minutes to 15 minutes, even as battery capacity increases. Reduced charging times allow for a more efficient use of the charging infrastructure.
- Consumer Charging Tendencies: The number of EV owners who can charge at home will impact public charging needs. A comparison of jurisdictions shows that Canadian home charging capacity is more in line with the United States than with Europe or China.
- Charging tendencies can also change over time. For example, early adopters are likely dominated by EV owners who have access to home charging. However, as the market matures, an increasing number of EV owners will not have access to home charging infrastructure. Without home charging, public charging infrastructure becomes more important.
- Market Penetration: When EV charging infrastructure is initially developed, enough geographic coverage is required to dispel range anxiety concerns. Chargers under the coverage scenario will tend to be underutilized until EV uptake increases. With this increased uptake, eventually these sites will reach capacity, and new sites and ports will be required. At this point, additional chargers will be needed to address capacity concerns.
2.2 Comparison with Actual Deployments
While there are a number of comparable studies that attempt to predict charging infrastructure needs based on adoption forecasts and analysis, we also have an increased opportunity to verify these studies with actual experience on the ground. Compared to 2018, we have the added advantage of several more years of real-world experience with charging infrastructure in a rapidly maturing global EV market. This offers an opportunity to validate the findings of these studies, given the complex dynamics tying together the multitude of factors at play.
The experience of Norway in particular represents a valuable opportunity for other regions to learn how the EV charging ecosystem must evolve over time to meet the needs of a growing EV fleet. While Norway’s EV market share had reached roughly 50% at the time of our 2018 study, EV market share in Norway in 2021 has approached and even exceeded 90% in some monthsFootnote 9. In terms of charging infrastructure capacity, the Norwegian EV Association reported just over 600,000 EVs in circulation as of September 2021, including over 435,000 BEVsFootnote 10. In January 2021, when accounting for dual-port CCS/CHAdeMO DCFC chargers that can charge only one vehicle at a time, they estimated a total of 3,200 unique fast-charge ports supporting a fleet of 330,000 BEVs at the time, representing a BEV-to-DCFC ratio of 103.
Meanwhile, EV owners in Norway reported a generally favourable experience with the charging infrastructure capacity in the country. The Institute of Transport Economics at the Norwegian Centre for Transportation Research has conducted extensive analysis of Norway’s charging ecosystem, including surveys of EV owner satisfaction and feedback on experience with lineups at fast charging stations. A 2019 study Footnote 11 indicated that between 41% and 54% of BEV owners reported experiencing fast charging queues depending on their location, with 12% to 18% reporting queues often or always:
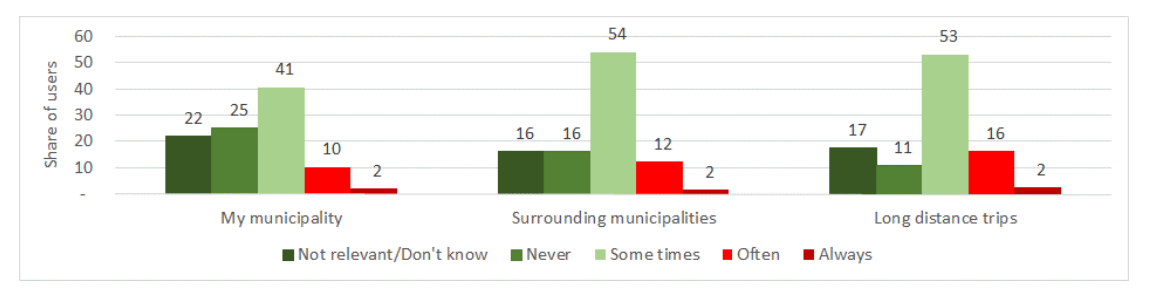
Figure 1: Where and how often BEV users (N = 1471) experience fast charging queues. Norwegian Centre for Transport Research, 2019.
Text version
Overview of where and how often EV drivers experience fast-charging queues in municipalities, chargers surrounding municipalities or on longer trips (e.g. long distance trips).
A 2020 analysis of fast charging on long-distance trips in inland NorwayFootnote 12 found similar results, with the majority of BEV owners expressing overall satisfaction with fast-charging infrastructure and waiting times.
We engaged with industry stakeholders that are actively deploying charging infrastructure in Canada and abroad, and monitoring EV driver experience with wait times, and we found that there is no obvious binary metric for determining whether or not charging infrastructure is adequate. EV drivers will likely have some tolerance for occasional congestion at charging sites, and this tolerance will likely vary depending on the type of user and type of trip, with EV early adopters generally being more accommodating. Besides the frequency of encountering congestion, the severity also needs to be considered. One stakeholder suggested that, while an occasional 10-minute wait is likely acceptable, wait times of an hour or more are obviously unfavourable and likely to lead to reluctance to own an EV.
In all cases, these stakeholders are balancing the need to manage infrastructure costs with the need to support peak travel times. Congestion at charging sites has typically occurred on peak travel days, such as during popular holiday weekends. A system designed to avoid congestion even on the busiest days would be oversized the rest of the year, whereas there is likely some tolerance for EV drivers regarding limited wait times on these busy days, and some flexibility to shift travel times to avoid congestion.
In all cases, the ability to measure and respond to actual EV user experience is crucial for fine-tuning the amount of charging infrastructure capacity and where it is targeted. We were told that, while our effort to predict Canada’s needs for charging infrastructure decades in advance is helpful for setting long-term targets and anticipating overall investment requirements, the actual needs for charging infrastructure will be determined through ongoing monitoring of infrastructure utilization and feedback from EV owners.
2.3 Overview of Updated Methodology
Based on our research, our proposed methodology largely follows the same overall steps as the methodology that we employed for our 2018 study:
- Forecast the projected number of EVs (BEVs and PHEVs) out to 2050 for all provinces and territories;
- Determine the number of EVs per public charger required for corridors (e.g., DCFCs along highways);
- Determine the number of EVs per public charger required to support community clusters (e.g., DCFC and L2 chargers in cities and towns); and
- Combine the number of EVs per public charger to determine the Canada-wide ratios and total infrastructure requirements based on EV forecasts.
While most of Dunsky’s methodology remains the same, there are several notable updates:
- Updated EV adoption forecast: Since the 2018 study, the federal government has accelerated its timeline to achieve a 100% ZEV market share for new LDV sales by 2035 instead of 2040. For this study, we relied on EV stock modeling provided by Transport Canada that assumed a 15% market share of new vehicle sales by 2025 and 60% market share by 2030. Based on our own modeling in other jurisdictions, an interim target of at least 60% is likely required to put Canada on a path towards achieving a 100% ZEV market share by the 2035 target date.
- Adopting Transport Canada ’s scenario represents an overall acceleration of the timeline for EV adoption and implementation of the required charging infrastructure compared to the timeline given in our 2018 study. That said, the acceleration is not as strong as initially anticipated because our 2018 study used an overly aggressive fleet turnover rate, achieving a 100% electric fleet by 2050 despite achieving 100% of new sales only by 2040. We believe that Transport Canada’s scenarios present a more realistic fleet turnover rate, barring any future policy developments intended to accelerate the retirement of ICE vehicles.
- Transport Canada ’s scenarios also include an estimate for the share of PHEVs in relation to BEVs that leans more towards PHEVs than the forecast developed for our 2018 study (e.g., the 2018 study estimated that BEVs would represent 88% of all ZEV sales in 2035, compared to 77% in Transport Canada’s scenario). As in our 2018 study, Transport Canada’s forecasts assume that Canada will achieve its ZEV adoption target based entirely on PHEV and BEV sales, implying that hydrogen fuel cell electric vehicle technology will play a negligible role in the LDV market.
- See Appendix A for more details on the forecasted EV fleet size.
- Updated projections for home charging access: While this analysis is focused on public charging, the needs for public charging infrastructure depend on the degree to which EV owners have the ability to charge at home. Improving home charging access through requirements for new constructions and retrofits is an important opportunity for enabling EV adoption. As such, we have included two scenarios with different levels of investment and policy support for home charging access, helping to highlight the degree to which such access influences the overall need for public charging infrastructure.
- Details of these two scenarios are provided in Appendix B.
- Updated assessment of L2 vs DCFC as a home charging substitute: For those without access to charging at home, public charging infrastructure can potentially serve as a substitute for residential charging. PHEV owners can rely on L2 charging infrastructure, either close to home (e.g., curbside charging) or close to work. Similarly, BEV owners without home charging can also rely on public L2 infrastructure, but BEV owners can also use DCFC infrastructure, such as a weekly visit to a fast-charging hub in a convenient location (e.g., grocery store). Our previous study included these options, but structured the calculations in a way that double-counted overall infrastructure needs between L2 and DCFC. In reality, BEV owners without home charging access will be served by a combination of L2 and DCFC infrastructure, and the total port requirements should reflect this combined impact. This update applies a downward pressure on the total L2 and DCFC port requirements, although the impact is relatively small given that this affects only BEV owners without home charging access.
- Two-port minimum for DCFC sites: Given observations in leading markets like Norway, and given the importance of reliability for charging infrastructure, we have adopted a best practice that was recommended by stakeholders in British Columbia: setting a minimum of two ports for all DCFC sites, regardless of modeled capacity requirements. This can help to ensure redundancy if ever there is a malfunction with a charging unit, and establishes a reasonable minimum starting point to avoid lineups as utilization increases. In more remote locations with relatively low traffic volumes, this may lead to an overall overbuild in charging capacity and low utilization of charging assets in the near term, but can ensure that these deployments are future-proofed for increasing EV traffic and that they offer redundancy for travellers on remote stretches.
The following sections describe our methodology in detail. In the conclusion, we present several opportunities for refining this approach through further research and analysis.
3.0 Highway Corridor Charging
While most BEV trips can be completed on a single charge, long-distance travel requires an extensive and convenient network of DCFC charging ports along highway corridors. To estimate corridor charging needs, two approaches should be considered:
- Initially, ensure that enough coverage is available to meet geographic connectivity needs;
- Later, ensure that enough capacity is available for the increase in charging volumes.
While early deployments of charging infrastructure are driven by a need to establish geographic connectivity, this analysis is intended to support projections over a long-term period (to 2050), and so we have focused our analysis on meeting capacity needs.
Aside from the updated EV adoption forecast, our approach for estimating charging needs on highway corridors is largely unchanged from the 2018 study, except for a two-port minimum requirement for all DCFC sites.
3.1 Methodology
Two different methodologies were used to estimate the number of DCFC ports and the number of DCFC sites along highway corridors in Canada.
For our projections, we assumed the National Highway System (NHS), which encompasses over 38,000 highway kilometres, would largely meet the corridor travel needs of Canadians. The NHS is broken down into three types of routes:
- Core Routes: interprovincial and international corridor routes;
- Feeder Routes: links to the Core routes from population and economic centres; and
- Northern and Remote Routes: links to the Core and Feeder routes that provide the primary means of access to northern or remote areas, economic activities and resources.
The total length associated with each of these route types across Canada is shown in Table 4.
Core Routes | Feeder Routes | Northern and Remote Routes | Total |
---|---|---|---|
27,722 | 4,411 | 5,916 | 38,049 |
For further clarification on the routes, refer to Figure 2.
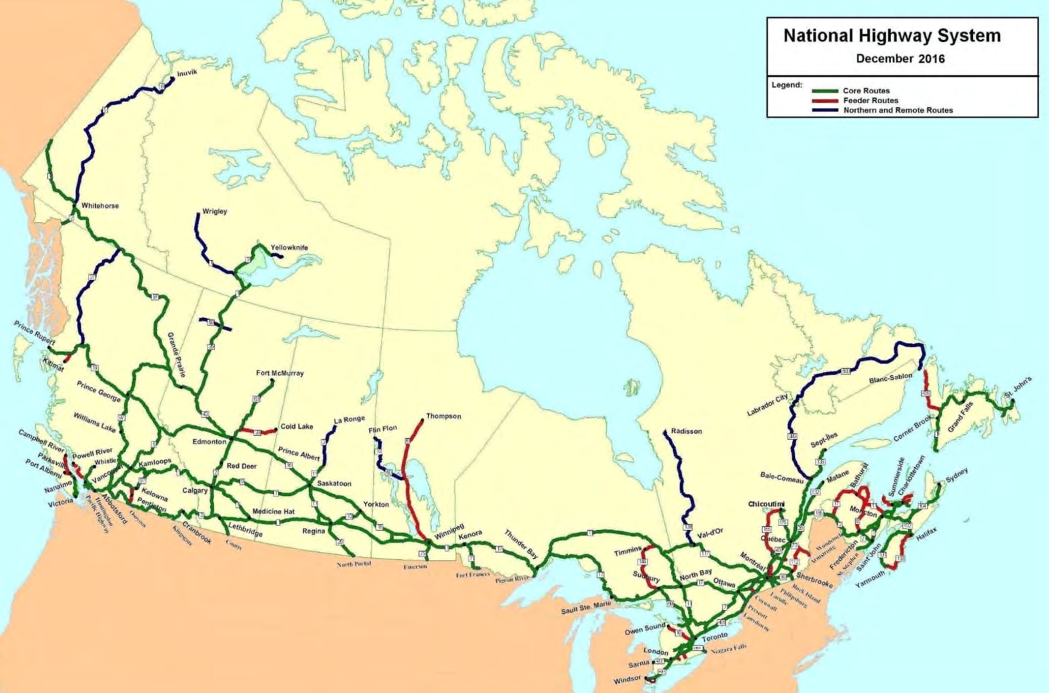
Figure 2: For this study, the National Highway System network was used to determine corridor charging needs. Footnote 14
Text version
A map showing the National Highway System road network in Canada.
The number of DCFC sites and ports along these corridors was then estimated using the following methodologies.
3.1.1 Estimating Fast-Charging Site Requirements
Figure 3 outlines the approach used to estimate the number of DCFC sites required across Canada on highway corridors.
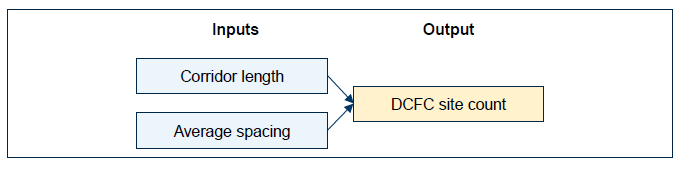
Figure 3: Methodology for estimating DCFC site count along corridors.
Text version
- Input
- Corridor length
- Average spacing
- Output
- DCFC site count
Many jurisdictions have opted for a simplified multiplier for average spacing to estimate the number of charging sites required along their primary corridors. With the total corridor length provided by the NHS, we have assumed a spacing of 65 km between sites. This is reflective of another NRCan study,Footnote 15 and is consistent with spacing requirements in other jurisdictions (as shown in Table 5).
Table 5: Corridor charging requirements in various jurisdictions.
Jurisdiction | Average Spacing | Minimum DCFC | Minimum Level 2 |
---|---|---|---|
NorwayFootnote 16 | 50 km | 2 ports | 2 ports |
FranceFootnote 17 | 50 km to 100 km | N/A | N/A |
Canada | 65 km | 2 ports | N/A |
United StatesFootnote 18 | 65 km to 160 km | N/A | N/A |
While a spacing of 65 km can ensure connectivity, enabling EV drivers to cover longer distances within the range capabilities of their vehicle, shorter spacing distances may benefit EV drivers from a convenience perspective. That said, the total port requirements would not be impacted by a tighter spacing of sites.
3.1.2 Estimating Fast-Charging Ports
Figure 4 outlines the approach used to estimate the number of DCFC ports required across Canada on highway corridors.
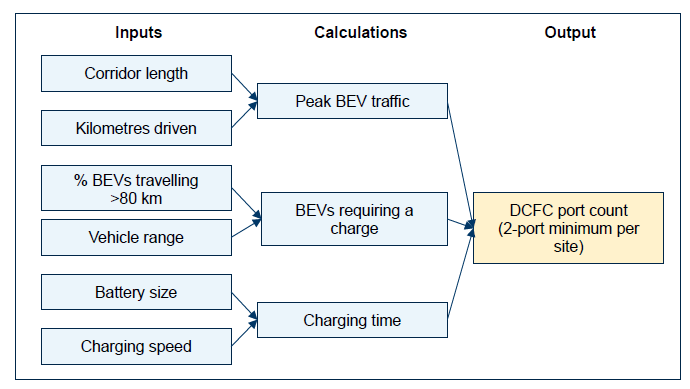
Figure 4: Methodology for estimating DCFC port count along corridors.
Text version
- Inputs
- Corridor length
- Kilometres driven
- Peak BEV traffic
- % BEVs travelling >80 km
- Vehicle range
- Battery size
- Charging speed
- Calculations
- BEVs requiring a charge
- Charging time
- Output
- DCFC port count
(2-port minimum per site)
- DCFC port count
The NHS provides the total kilometres travelled along its various networks for an annual period. These distances were used to estimate the average number of trips per hour across the corridors. The total kilometres travelled in a province for a route category was divided by an average trip length of 43 kilometresFootnote 19 and the total number of hours in a year to determine the average number of trips per hour. Once the average number of trips per hour was determined, the peak traffic conditions (for all Light-Duty Vehicles [LDVs]) were estimated using a multiplying factor of 2.3 times the average number of trips per hour.Footnote 20 By estimating the ratio of BEVs to LDVs (based on fleet size, as described in Appendix A), LDV peak traffic was then converted to BEV peak traffic.
To determine the number of BEVs requiring a charge, an adjustment factor was estimated based on trip distance, charging time and battery capacity. Charging time was deemed to be a function of the average battery capacity (kWh) and the average charging power (kW). This adjustment factor ranges from approximately 5% in 2020 to just over 1% in 2050. This analysis was performed at the provincial level, and aggregated to provide national requirements.
New for 2021: A minimum requirement of two ports per DCFC site was applied to maximize redundancy and reliability.
3.2 Highway Corridor Results
Based on our analysis, the projected DCFC port requirements to provide sufficient capacity across Canada’s National Highway System over time are outlined in Table 6.
2025 | 2030 | 2035 | 2040 | 2045 | 2050 | |
---|---|---|---|---|---|---|
DCFC Ports | 1,300 | 2,100 | 3,700 | 5,700 | 7,200 | 8,100 |
BEV/DCFC | 700 | 1,700 | 2,500 | 2,800 | 3,000 | 3,200 |
It is important to note that the charging port requirements for highway corridors are not impacted by home charging access, and therefore results do not vary according to the retrofit scenarios.
To ensure adequate geographic coverage, a minimum of 585 sites would be required across the identified corridors. If we assume a minimum of two ports per site (as we recommend for the sake of redundancy and reliability), the minimum coverage requirement would indicate a need for a minimum of 1,170 ports. Based on the above capacity projections and assuming that stations are constructed to meet coverage demands, on average, capacity requirements will exceed this minimum requirement. That said, site-specific capacity requirements are likely to vary significantly based on traffic volumes on specific corridors. This may mean that lower traffic volume corridors will be oversized in terms of charging capacity due to our minimum two-port requirement.
A more detailed analysis (per corridor segment using geospatial traffic analysis) is needed to fully understand local charging capacity needs. Rather than assessing the needs of each corridor, the above results provide high-level guidance to satisfy charging requirements on a national scale across highway corridors.
4.0 Community Cluster Charging
While charging on highway corridors is often the dominant topic of conversation, EVs will also benefit from having access to public charging infrastructure in densely populated areas. A diversified charging infrastructure, incorporating both fast- and slower-charging infrastructure, will allow EV owners to select the optimal charging methods for their individual needs.
As with corridor charging, it is important to consider two aspects of the charging infrastructure:
- initially, ensure that enough coverage is available to meet geographic connectivity needs;
- later, ensure that enough capacity is available for the increase in charging volumes.
Again, we have focused our analysis on meeting capacity needs. Due to the greater geographic density of the clusters that we aim to populate, geographic coverage should be easier to meet than coverage on corridors.
Given the important interdependency between public charging and residential charging, our methodology has been updated to incorporate two scenarios with differing levels of home charging access. Our methodology also incorporates the same minimum two-port requirement for all DCFC sites as in the highway corridor methodology.
4.1 Methodology
For clusters, three different approaches were used to estimate the number of:
- DCFC sites (i.e., minimum number required for geographic coverage);
- DCFC ports (including a minimum of two (2) ports per site); and
- Level 2 ports.
The calculations are based on clusters defined as areas with populations of over 1,000 people. Three sets of clusters were defined and segmented by province and territory. Additional information about these cluster types is presented in Table 7.
Large Urban Cluster | Medium Cluster | Small Cluster | |
---|---|---|---|
Definition (Population) | >100,000 | 100,000–30,000 | 30,000–1,000 |
Count | 31 | 56 | 923 |
Total Population (in millions) | 20.9 | 3.1 | 4.5 |
Total Land Area (in km2) | 9,487 | 2,432 | 4,892 |
Rural areas―those with populations of fewer than 1,000 people―were not included when estimating future charging needs, as it was assumed that their needs would be covered by the corridor and cluster requirements.
4.1.1 Estimating DCFC Sites
Figure 5 outlines the approach used to estimate the number of DCFC sites required in clusters.
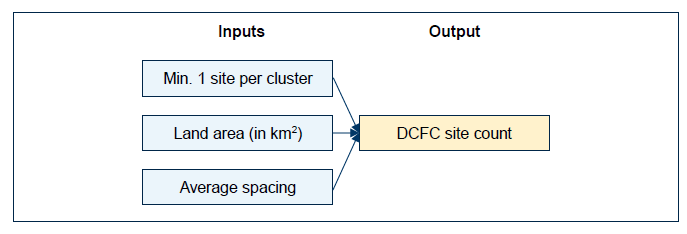
Figure 5: Methodology for estimating DCFC site count in clusters.
Text version
- Output
- Min. 1 site per cluster
- Land area (in km2)
- Average spacing
- Inputs
- DCFC site count
A two-step process was used to estimate the number of DCFC sites required in all Canadian clusters. First, it was assumed that at least one site would be required for each of the 1,010 large, medium and small clusters. Then, to ensure a sufficient number of sites in larger clusters, the size of the cluster was considered. In the United States, an analysis was performed to estimate geographic coverage requirements in cities. The study determined that, in order to allow a driver to never be more than 5 linear kilometres away from a charging site, 22 sites would be required per 1,000 km2.Footnote 22 We have used this assumption to estimate the minimum number of DCFC sites required to ensure adequate geographic coverage within clusters.
4.1.2 Estimating DCFC Ports
Figure 6 outlines the approach used to estimate the number of DCFC ports required in clusters across Canada.
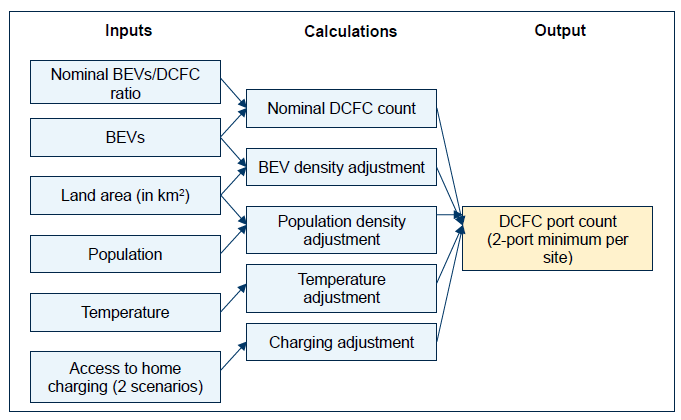
Figure 6: Methodology used for estimating DCFC port count in clusters (population >1,000 people).
Text verison
- Inputs
- Nominal BEVs/DCFC ratio
- BEVs
- Nominal DCFC count
- Land area (in km2)
- Population
- Temperature
- Access to home charging (2 scenarios)
- Calculations
- Population density adjustment
- Charging adjustment
- Temperature adjustment
- BEV density adjustment
- Output
- DCFC port count
(2-port minimum per site)
- DCFC port count
A nominal ratio of 250 BEVs per DCFC port was used as a starting point, based on an estimate from the United States.Footnote 23 The estimate assumes a home-dominant charging pattern. The following adjustment factors were deemed to impact charging infrastructure requirements:Footnote 24
- BEV Density Adjustment: As the density of BEVs in an area increases, the ratio of BEVs per DCFC will also increase. For example, in a given area, more chargers will need to be added when moving from 100 to 200 EVs, then when moving from 1,000 to 1,100 EVs. The number of BEVs per province and per cluster type was estimated in order to apply this adjustment factor.
- Population Density Adjustment: As the population density in an area increases, there is also an expectation that the ratio of BEVs per DCFC will increase. The population density per province and per cluster type was used to apply this adjustment factor.
- Temperature Adjustment: Temperature can impact the range and charging speed for EVs. In areas where temperatures are often too cold or too hot, additional charging infrastructure will be needed. To estimate this temperature adjustment, a weighted average adjustment factor was determined based on the average monthly temperature in each province.
- Home Charging Adjustment: Not all EV owners will have access to at-home charging infrastructure. This can be particularly true for owners living in Multi-Unit Residential Buildings (MURBs). To address this impact, we adjusted the nominal ratio by estimating the percentage of the population who did not have access to home charging and who were therefore required to have access to a charging level 10 times the nominal ratio (i.e., 25 BEVs per DCFC port for owners without access).
- New for 2021: Whereas our 2018 study included a single time series forecast for total home charging access, this study includes two scenarios with differing levels of home charging access to highlight the impact that this has on overall public charging demand. See Appendix B for more details.
Once these adjustment factors had been determined, the estimated number of BEVs was divided by the nominal ratio multiplied by the adjustment factors to determine the total amount of DCFC ports required in clusters.
New for 2021: a minimum requirement of two ports per site was applied for all DCFC sites.
4.1.3 Estimating Level 2 Ports
In clusters, a similar methodology was used to estimate the required Level 2 ports as was used for the DCFC ports. The primary exception is that the nominal ratio used for Level 2 ports varies based on the projected ratio of BEVs to PHEVs.Footnote 25 Regardless of this, the same process was used to account for the adjustment factors.
4.2 Community Charging Results
Based on our analysis, the projected DCFC and L2 ports in clusters are outlined in Table 8.
2025 | 2030 | 2035 | 2040 | 2045 | 2050 | ||
---|---|---|---|---|---|---|---|
Scenario 1: High access to home charging |
DCFC Ports | 3,000 | 11,600 | 28,200 | 44,500 | 55,500 | 60,900 |
BEVs / DCFC | 240 | 300 | 340 | 370 | 400 | 430 | |
Level 2 Ports | 48,000 | 181,000 | 410,000 | 593,000 | 673,000 | 658,000 | |
EVs / Level 2 | 21 | 26 | 30 | 35 | 40 | 47 | |
Scenario 2: Low access to home charging |
DCFC Ports | 3,000 | 12,000 | 30,000 | 49,400 | 65,300 | 76,800 |
BEVs / DCFC | 310 | 300 | 310 | 330 | 330 | 340 | |
Level 2 Ports | 49,000 | 186,000 | 436,000 | 659,000 | 791,000 | 830,000 | |
EVs / Level 2 | 21 | 25 | 28 | 31 | 34 | 37 |
To ensure adequate geographic coverage across all Canadian cities with populations greater than 1,000 people, a minimum of 1,194 cumulative DCFC sites are required (e.g., a small cluster could require one (1) site, while a large urban cluster could require seven (7) sites). If we assume a minimum of two ports per DCFC site (as we recommend for the sake of redundancy and reliability), the minimum coverage requirement would indicate a need for a minimum of 2,388 DCFC ports. This means that, by 2025, the charging capacity rather than geographic coverage would already be the driving factor determining the total need for ports.
5.0 Canada-wide Charging Requirements
Canada-wide public charging requirements are determined by combining the corridor and cluster projections.
5.1 Results
Table 9 outlines the total public charging infrastructure needs and EV-to-charger ratios for Canada, combining both highway corridor and community cluster infrastructure, and including assumptions related to home charging access under each scenario.
2025 | 2030 | 2035 | 2040 | 2045 | 2050 | ||
---|---|---|---|---|---|---|---|
Scenario 1: High access to home charging |
Public DCFC | 4,300 | 13,800 | 32,000 | 50,200 | 62,700 | 69,000 |
Public L2 | 48,000 | 181,000 | 410,000 | 593,000 | 673,000 | 658,000 | |
Total Public Ports | 52,000 | 195,000 | 442,000 | 643,000 | 736,000 | 727,000 | |
Total MURB Ports | 515,000 | 1,302,000 | 2,189,000 | 3,191,000 | 4,326,000 | 5,610,000 | |
EVs / Level 2 | 21 | 26 | 30 | 35 | 40 | 47 | |
BEVs / DCFC | 180 | 250 | 300 | 330 | 350 | 380 | |
EVs / Public Port | 20 | 24 | 28 | 32 | 37 | 43 | |
EVs / Total Ports | 2 | 3 | 5 | 5 | 5 | 5 | |
Scenario 2: Low access to home charging |
Total DCFC | 4,300 | 14,100 | 33,700 | 55,100 | 72,500 | 84,900 |
Total L2 | 49,000 | 186,000 | 436,000 | 659,000 | 791,000 | 830,000 | |
Total Ports | 53,000 | 201,000 | 469,000 | 714,000 | 864,000 | 914,000 | |
Total MURB Ports | 46,000 | 152,000 | 499,000 | 886,000 | 1,318,000 | 1,799,000 | |
EVs / Level 2 | 21 | 25 | 28 | 31 | 34 | 37 | |
BEVs / DCFC | 170 | 240 | 280 | 300 | 300 | 310 | |
EVs / Public Ports | 20 | 23 | 26 | 29 | 31 | 34 | |
EVs / Total Ports | 11 | 14 | 13 | 13 | 13 | 12 |
Note: Totals may not reflect the sum of individual line items due to rounding.
By way of reference, Table 10 below shows the results obtained in the 2018 study.
2025 | 2030 | 2035 | 2040 | 2045 | 2050 | ||
---|---|---|---|---|---|---|---|
2018 Study Results | Total DCFC | 3,800 | 11,600 | 33,000 | 50,800 | 63,700 | 77,600 |
Total L2 | 42,000 | 101,000 | 240,000 | 361,000 | 443,000 | 536,000 | |
Total Ports | 45,000 | 112,000 | 273,000 | 412,000 | 506,000 | 614,000 | |
EVs / Level 2 | 22 | 31 | 41 | 46 | 53 | 56 | |
BEVs / DCFC | 180 | 220 | 260 | 290 | 330 | 350 | |
EVs / Ports | 20 | 27 | 36 | 41 | 46 | 49 |
Across both scenarios, our updated approach, including the accelerated timeline for EV adoption aligning with Canada’s new EV adoption targets, highlights a need for a significant acceleration of charging infrastructure deployment in the near term (2025‒2030). In the longer term, the results are more mixed compared to our 2018 study depending on the scenario, with an increase in overall L2 requirements in all scenarios, and either an increase or decrease in DCFC requirements depending on the level of home charging access.
6.0 Conclusions
The findings of our updated analysis highlight the need for significant ongoing investment in public charging infrastructure across Canada. While the results are largely consistent with our previous analysis, we note a few key findings below:
- Compared to our 2018 study, we see a need for a significant acceleration in charging infrastructure deployment over the next five to ten years in order to support the federal government’s target of achieving 100% EV market share of new light-duty vehicle sales by 2035. By 2025, we see a need for 4,300 DCFC ports across Canada, a significant increase from the 3,800 we predicted in our 2018 study and from the roughly 3,000 DCFC ports currently installed as of November 2021Footnote 26.
- Our approach based on optimal EV-to-charger ratios helps to highlight the need for ongoing investment to keep pace with a growing EV population. In the very long term, our overall estimate for public charging infrastructure needs across Canada is up to 84,900 DCFC ports and 830,000 L2 ports by 2050. If we assume an average of $150,000 total cost per DCFC port and $8,000 per L2 port, this would represent a total investment of approximately $20 billion over the next three decades. But while this analysis quantifies the total infrastructure needed to meet Canada’s EV adoption targets, this study does not assess what portion of this infrastructure would require support from the federal government. The federal government is currently leveraging significant co-investment from other actors in the EV charging ecosystem, and we can expect the overall proportion of federal investment in charging infrastructure to decline as this ecosystem becomes increasingly competitive and attracts investment from other actors.
- As we saw in our 2018 study, the primary driver for the number of charging ports is capacity requirements within community clusters. While infrastructure along highway corridors is essential to ensure connectivity, the long distances between populated areas in Canada mean that many of these corridors do not serve high volumes. Clusters, including towns and cities with a population greater than 1,000 people, represent the bulk of the needs for charging infrastructure deployment.
- The charging infrastructure within community clusters is especially important for those without access to charging at home. The results from our two scenarios for different levels of home charging access highlight the benefits of taking aggressive actions to improve home charging access. Charging at home overnight is the most convenient option for EV owners and can also be the most cost-effective option when charging infrastructure is deployed at scale and incorporated into new buildings during construction. The additional investment required in public charging infrastructure in the low-retrofit scenario corresponds to 15,900 DCFC ports and 172,000 L2 ports. Assuming $150,000 per DCFC port and $8,000 per L2 port, this would represent $3.8 billion of investment. We estimate that this would be roughly double the cost of the additional 900,000 apartment/condo parking stall retrofits included in the high-retrofit scenario (assuming $1,200 per stall for comprehensive full-building EV-ready retrofits and $1,000 per L2 charger installed). This means that ongoing efforts by the federal government to retrofit existing buildings and to ensure that new buildings are designed with EV charging in mind will lead to significant cost savings through reduced needs for public charging, while also making EV ownership more convenient for a broader range of Canadian households.
- For households that do not have access to charging at home, public charging infrastructure can potentially serve as a substitute. While this is typically less convenient and more expensive than charging at home, it may be the only option for some households, including those without any off-street parking at all, and those in multi-unit residential buildings that have not yet added charging infrastructure. Our updated analysis includes a balance of L2 and DCFC infrastructure in population centres. The actual relative importance of DCFC and L2 infrastructure will depend on a number of factors, including the following:
- EV owner’s convenience (e.g., a weekly trip to a DCFC hub at a grocery store vs more frequent use of curbside or other L2 charging stations close to home);
- charging costs (DCFC usage fees are typically much higher than for L2 charging);
- cold weather considerations (e.g., more regular charging at L2 stations may be preferable to maintain optimal battery temperatures, whereas fast charging performance can be significantly slower due to cold battery temperatures in a vehicle that has been left unplugged in cold weather for several days);
- infrastructure scalability (large-scale L2 deployments on city streets can be challenging and may lead to conflicts with other street users such as pedestrians).
Overall, the results presented in this study represent our best estimate of what will be needed to support Canada’s ZEV adoption targets over the coming decades. There is significant uncertainty in a number of aspects of the analysis as discussed above, and unforeseen technology advancements could significantly alter Canada’s ZEV infrastructure pathway. For example, further advancements in fast charging capabilities and battery thermal management could further reduce fast charging times beyond what we anticipated in this analysis, potentially reducing the total number of DCFC ports required and reducing overall reliance on Level 2 charging. Ultimately, investments in charging infrastructure can grow over time in response to anticipated demand on a shorter timescale than the decades-long outlook presented here. But laying out a roadmap for future infrastructure needs based on our best understanding today is essential to guide near-term policies and investments, and to identify gaps in our understanding that warrant further analysis.
6.1 Recommendations for Further Analysis
This report presents our findings from a brief review of our previous analysis, recent studies, and input from industry stakeholders. The topic of infrastructure deployment is complex and evolving, and ongoing federal investment will benefit from further analysis on a range of topics, including the following:
- Detailed geographic modeling: While this study summarizes Canadian charging needs at a high level, further analysis is required to understand the localized impacts of EV adoption on charging needs, such as analysis of specific highway corridors and their peak and average travel volumes.
- Study of preferred charging options in urban centres: As described above, the best approaches for charging infrastructure depend on a wide range of factors, including the preferences of current and future EV drivers. Further research could help to refine our understanding of which charging approaches are the best fit for different user groups (e.g., curbside Level 2 charging vs neighbourhood DCFC hubs for garage orphans). Research could also help to inform and optimize urban charging strategies accordingly.
- Charging infrastructure needs for medium- and heavy-duty vehicles: This analysis focused entirely on light-duty vehicles. Medium- and heavy-duty vehicles are increasingly within reach of EV technologies. Their needs for charging infrastructure will vary significantly depending on the vehicle type and application, but some aspects will show parallels with this analysis (e.g., highway corridor charging for long-haul trucks).
- Assessment of the business case for private investment: This analysis quantifies the total infrastructure needed to meet Canada’s EV adoption targets, but does not assess what portion of this infrastructure would require support from the federal government. While the business case for public charging infrastructure can be challenging due to the prevalence of residential charging, increased utilization over time thanks to a growing EV population should improve charging infrastructure economics in the coming years. Analysis of the potential profitability of different types of charging infrastructure in different contexts could help the federal government and other stakeholders to focus their efforts to encourage private investment as much as possible, while filling gaps in areas that are likely to be underserved by private investments.
Appendix A – Estimating Fleet Size
Transport Canada and NRCan provided Dunsky with scenarios for EV adoption based on the federal target of achieving 100% market share of new LDV sales by 2035. While interim targets have not yet been established, we used a scenario developed by Transport Canada that achieves a market share of 15% by 2025 and 60% by 2030.
2020 | 2025 | 2030 | 2035 | 2040 | 2045 | 2050 | |
---|---|---|---|---|---|---|---|
BEVs | 121,403 | 724,994 | 3,455,738 | 9,567,618 | 16,461,931 | 22,063,526 | 26,129,854 |
PHEVs | 81,747 | 294,015 | 1,177,021 | 2,799,204 | 4,189,862 | 4,869,634 | 4,880,810 |
EVs | 203,150 | 1,019,009 | 4,632,759 | 12,366,822 | 20,651,792 | 26,933,160 | 31,010,664 |
BEV (%) | 60% | 71% | 75% | 77% | 80% | 82% | 84% |
PHEV (%) | 40% | 29% | 25% | 23% | 20% | 18% | 16% |
% of Fleet | 0.8% | 3.8% | 16% | 40% | 63% | 80% | 90% |
As we did for our 2018 study, we further broke down these estimates by province based on a gradual progression from today’s provincial breakdown of EV adoption to a more uniform distribution across all provinces by 2050.
Appendix B – Estimating Home Charging
To forecast access to home charging, building growth rate trends were taken from the 2011 and 2016 census. The rate of new construction (as a percentage of existing buildings) was developed using the Canada Mortgage and Housing Corporation (CMHC) publication “Housing Starts, Completions and Units Under Construction.” For the purposes of this study, “persons who have access to home charging” are defined as those who have charging at home, or those who can install a Level 2 charger without significant barriers (similar to a typical single-family home with off-street parking). The following assumptions were made for the high- and low-retrofit scenarios:
- High-Retrofit Scenario: 1,000,000 Multi-Unit Residential Building (MURB) stall retrofits by 2030 and a 100% EV readiness requirement for new construction introduced by 2025.
- Low-Retrofit Scenario: 100,000 Multi-Unit Residential Building (MURB) stall retrofits by 2030 and a 100% EV readiness requirement for new construction introduced by 2030.
Table 12 outlines the evolving home charging access as a result of the above scenarios.
2025 | 2030 | 2035 | 2040 | 2045 | 2050 | ||
High-Retrofit Scenario | % of Population Living in Single-Family Dwellings | 68% | 66% | 64% | 63% | 61% | 59% |
% of Single-Family Occupants with Access to Home Charging | 85% | 85% | 85% | 85% | 85% | 85% | |
% of Population Living in Multi-Family Dwellings | 32% | 34% | 36% | 37% | 39% | 41% | |
% of Multi-Family Dwellings with Access to Home Charging | 15% | 34% | 52% | 68% | 83% | 97% | |
Low-Retrofit Scenario | % of Population Living in Single-Family Dwellings | 68% | 66% | 64% | 63% | 61% | 59% |
% of Single-Family Occupants Access to Home Charging | 85% | 85% | 85% | 85% | 85% | 85% | |
% of Population Living in Multi-Family Dwellings | 32% | 34% | 36% | 37% | 39% | 41% | |
% of Multi-Family Dwellings with Access to Home Charging | 2% | 4% | 11% | 18% | 24% | 30% |
This report was prepared by Dunsky Energy + Climate Advisors. It represents our professional judgment based on data and information available at the time when the work was conducted. Dunsky makes no warranties or representations, expressed or implied, in relation to the data, information, findings and recommendations contained in this report or in related work products.
Page details
- Date modified: