Energy Efficiency Reference Guide Compressed Air
Table of contents
- How This Guide Is Organized
- Purpose of this Reference Guide
- What is Compressed Air?
- Introduction to Compressed Air Systems
- Air Compressor Types and Controls
- Compressed Air Auxiliary Equipment
- Uses and Misuses of Compressed Air
- So You Want to Perform a Compressed Air System Assessment?
- Proven Energy Efficiency measures
- Maintaining Your Compressed Air System
- Before and After Case Examples
- Next Steps
- Glossary
- Literature and Web references
- Quick Tips to Optimize Compressed Air Systems
How This Guide is Organized
This handbook is divided into 16 main sections. The overarching theme is to help you to understand, and implement a program to improve the energy efficiency of compressed air systems. The handbook covers a mixture of theory and practice. It also includes some general case examples, glossary and references. The last chapter contains tips for those who want to optimize a compressed air system very quickly.
Theme | See Chapter |
Introduction to the Guidebook | Purpose of this Reference Guide What is Compressed Air? |
Compressed Air Systems and Components | Introduction To Compressed Air Systems Air Compressor Types and Controls Compressed Air Auxiliary Equipment |
Reducing Energy Consumption | Uses and Misuses of Compressed Air |
Compressed Air Assessments | So You Want to Perform a Compressed Air System Assessment? |
Ongoing Optimization | Proven Energy Efficiency Improvements Maintaining Compressed Air System |
Case Examples | Before and After Case Examples Next Steps |
Reference | Glossary Literature and Web References Useful Measurement and Conversion Factors |
Implementing a Plan | Quick Tips to Optimize Compressed Air Systems |
The handbook contains boxed highlighted sections with compressed air energy savings and operations tips
Energy Savings ideas are generally shown within a box with a grey background.
Key points and tips are enclosed in a box with a blue background.
Purpose of this Reference Guide
This guidebook addresses typical compressed air systems common to most small and medium manufacturing facilities. It covers common compressed air design and operating problems. It is intended to provide you with guideposts about your compressed air system, as well as things to think about as you begin or continue to optimize your compressed air system for peak performance.
You are probably wondering why you should read or refer to this reference guide. You may find this guidebook to be helpful should one or more of the following situations apply to you.
- Utility bills are rising, and your boss wants you to reduce energy costs at your facility.
- You are faced with replacing an old compressor or expanding your compressed air system.
- You know you need to “fix” your compressed air system, but are not sure where to start, what to look for, or where to go for the right information.
- Production is suffering due to excessive downtime or insufficient pressure from your air compressor system.
- You need to provide a sound solution to an internal or external customer who is experiencing difficulties with their compressed air system.
If these situations apply to your circumstances, you probably have one of the following roles:
- A production or maintenance manager looking for ways to save money and boost productivity.
- A utility representative with a mandate to help customers to become more energy efficient.
- An air compressor equipment or service business development representative seeking ways to help your customers to solve an air-related problem.
- A student or trainee who wants to learn about air compressor systems.
Why Compressed Air?
Compressed air is not free, but unfortunately it is often treated as such. You should be aware that compressed air is expensive to produce, and is likely consuming a significant slice of your energy dollar.
If you are like most industrial manufacturing or processing businesses, chances are that you have a compressed air system. Your air compressor system may be located somewhere out of sight and out of mind at your facility. You may not know what it costs to operate and maintain.
In addition to system operating costs, there are system reliability and performance issues to be concerned with, as well as the quality of the compressed air. These direct and indirect costs can be determined by measuring and baselining your system.
Compressed air is a controllable cost, and this guidebook will help you to identify some common ways to reduce the energy, maintenance and capital costs associated with owning and operating your compressed air system.
What this Guidebook is and is Not
This guidebook was written to help you to become aware of the costs of compressed air, and to point you in the right direction in helping you to reduce these costs. Energy efficiency best practices and tips are suggested and emphasized.
This guidebook addresses the typical compressed air systems common to most small and medium manufacturing facilities. It covers common compressed air design and operating problems.
It is intended to provide you with guideposts about your compressed air system, as well as things to think about as you begin or continue to optimize your compressed air system for peak performance.
This guidebook is general in nature and does not address each and every possible problem and solution that is associated with compressed air systems. It is not a design guide for new or expanded air compressor systems. This guidebook does not, and is not intended to replace equipment manuals, or maintenance procedures.
Readers are cautioned to use proper health, safety, and lockout procedures, and to follow equipment operating and maintenance manuals before, during and after any modifications, work or testing related to compressed air systems. Failure to follow proper and safe procedures and codes could result in serious injury, loss of life, property damage, production upsets and other risks.
What is Compressed Air?
Compressed air is a form of stored energy that is used to operate machinery, equipment, or processes. Compressed air is used in most manufacturing and some service industries, often where it is impractical or hazardous to use electrical energy directly to supply power to tools and equipment.
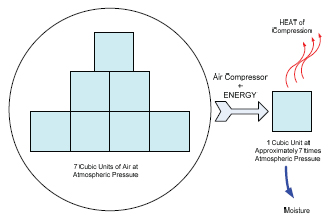
Figure 1 – Conversion of Atmospheric Air into Compressed Air
Text version
Figure 1 – Conversion of Atmospheric Air into Compressed Air
Diagram showing 7 cubic units of air being compressed by an air compressor, which requires energy, and producing 1 cubic unit of compressed air at aproximately 7 times atmospheric pressure, plus heat of compression and moisture.
Powered by electricity, a typical air compressor takes approximately 7 volumes of air at atmospheric conditions, and squeezes it into 1 volume at elevated pressure (about 100 psig, [7 bar]). The resulting high pressure air is distributed to equipment or tools where it releases useful energy to the operating tool or equipment as it is expanded back to atmospheric pressure.
In the compression process, and the subsequent cooling of the air to ambient temperatures, heat and moisture, are released as illustrated in Figure 1.
Recovered heat from the air compressor can potentially be used as an energy efficiency measure for other processes, such as space and water heating.
Depending on the application, excessive moisture in compressed air needs to be managed as it can cause problems with piping (corrosion) and end use equipment.
Compressed Air Costs
This section will help you to understand how much it costs to produce and use compressed air.
Over the first ten years of life of a typical air cooled compressor (see Figure 2), with two shift operation, the operating cost (electricity and maintenance) will equal about 88% of the total lifetime cost. The cost of the original equipment and installation will account for the remaining 12%.
As energy accounts for about 76% of the overall lifetime operating cost, it is very important to design and purchase the most efficient components for your compressed air system. It is recommended to make purchase decisions on the overall expected lifetime operating costs, and NOT just on the initial cost of the equipment.
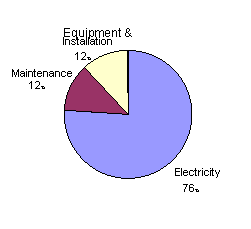
Figure 2 - Typical Lifetime Ownership Cost of Compressed Air Systems
Text version
Figure 2 - Typical Lifetime Ownership Cost of Compressed Air Systems
Pie chart showing 76 percent of cost of ownership is for electricity; 12 percent is for maintenance; and 12 percent is for equipment and installation.
Figure 3 illustrates the typical losses associated with producing and distributing compressed air. Assuming 100 HP energy input, approximately 91 HP ends up as losses, and only 9 HP as useful work. In other words, about 90% of the energy to produce and distribute compressed air is typically lost.
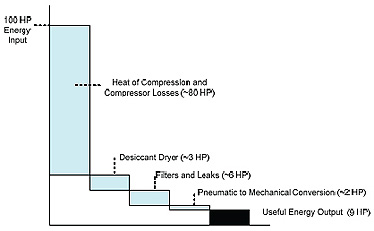
Figure 3 - Compressed Air Energy Input and Useful Energy Output
Text version
Figure 3 - Compressed Air Energy Input and Useful Energy Output
Bar chart showing the typical losses associated with producing and distributing compressed air starting with 100 HP energy input. The first bar shows that aproximately 80 HP is lost as heat of compression and compressor losses; the second bar shows that aproximately 3 HP are lost to the dessicant dryer; the third bar shows that aproximately 6 HP are lost to filters and leaks; the fourth bar shows that aproximately 2 HP are lost to pneumatic to mechanical conversion; the fifth bar shows that the useful energy output is 9 HP.
Always question if compressed air is the most appropriate power source for an end use application. In many cases, you would be better off to use a direct drive electric tool instead of a compressed air driven one.
Some industrial compressors are cooled with water. In such cases, the additional sewer and water charges, chilled water system operating costs, pumping costs and chemical treatment need to be evaluated. Figure 4 is a simplified table to provide you with an indication of the electricity costs associated with one, two and three shift operations at a typical industrial facility. The table also shows the approximate electricity cost for 10, 15, 25, 50 and 100 HP-sized air compressors. The table assumes the compressor average loading is 65% of full load.
The table in Figure 4 uses a blended (energy and demand) electricity rate of $0.10 per kWh. Depending on your local electricity tariff rates, you will need to multiply the values in Figure 4 by your inpidual electricity rate (dollars per kWh) and then multiply by 10 to derive your annual electricity costs. Contact your local utility or compressed air service provider for help to determine your own blended electricity rate.
1 Shift (2,250 Hrs) |
2 Shifts (4,250 Hrs) |
3 Shifts (8,400 Hrs) |
|
---|---|---|---|
10 HP | $1,720 | $3,250 | $6,430 |
15 HP | $2,580 | $4,880 | $9,640 |
25 HP | $4,300 | $8,130 | $16,060 |
50 HP | $8,600 | $17,260 | $32,130 |
100 HP | $17,120 | $32,330 | $63,900 |
Add approximately 25% to the annual compressed air electricity cost to account for maintenance and life cycle capital cost (purchase price) of compressed air system. For example, a 50 HP compressor operating for one shift would cost approximately $8,600 for electricity alone, and approximately an additional $2,150 for maintenance and capital costs, for a total of $10,750.
Most facilities can easily save 10-20% of their compressed air energy costs through routine maintenance such as the fixing of air leaks, lowering air pressure, and replacing clogged filters. Even higher savings numbers can be gained by choosing better compressor control, adding storage receiver capacity, and upgrading air dryers and filters.
Using the information in Figure 4, what is the potential to save money and energy at your facility?
Introduction to Compressed Air Systems
Compressed air systems consist of a number of major subsystems and components. Compressed air systems can be subpided into the Supply and Demand side.
The Supply side includes compressors, air treatment and primary storage. A properly managed supply side will result in clean, dry, stable air being delivered at the appropriate pressure in a dependable, cost effective manner. Major compressed air supply subsystems typically include the air intake, air compressor (fixed speed and/or variable speed), aftercooler, motor, controls, treatment equipment and accessories.
Controls serve to adjust the amount of compressed air being produced to maintain constant system pressure and manage the interaction between system components. Air filters and air dryers remove moisture, oil and contaminants from the compressed air. Compressed air storage (wet and dry receivers) can also be used to improve system efficiency and stability. Accumulated water is manually or automatically discharged through drains. Optional pressure controllers are used to maintain a constant pressure at an end use device.
The Demand side includes distribution piping, secondary storage and end use equipment. A properly managed demand side minimizes pressure differentials, reduces wasted air from leakage and drainage and utilizes compressed air for appropriate applications. Distribution piping systems transport compressed air from the air compressor to the end use point where it is required. Compressed air storage receivers on the demand side can also be used to improve system pressure stability.
As a rule of thumb, for every horsepower (HP) in the nameplate capacity, the air compressor will produce approximately 4 standard cubic feet per minute (scfm).
A simplified diagram illustrating how some of the major components are connected is shown in Figure 5.
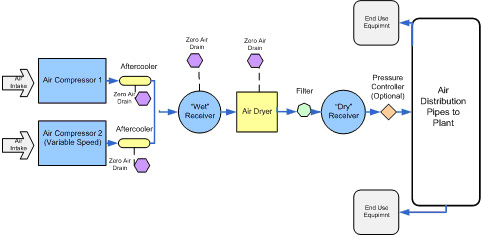
Figure 5 - Common Air Compressor System Components
Text version
Figure 5 - Common Air Compressor System Components
diagram showing air compressors, aftercoolers, "wet" receiver, air dryer, filter, "dry" receiver, pressure controller, air distribution pipes, and end use equipment.
Compressed Air Use
Compressed air is used for a perse range of commercial and industrial applications. As it is widely employed throughout industry, it is sometimes considered to be the “fourth utility” at many facilities.
In many facilities, compressed air systems are the least energy efficient of all equipment. There is a tremendous potential to implement compressed air energy efficiency practices.
It has been common practice in the past to make decisions about compressed air equipment and the end uses based on a first cost notion. Ongoing energy, productivity and maintenance costs need to be considered for optimal systems. In other words, best practice calls for decisions to be based on the life cycle cost of the compressed air system and components.
Improving and maintaining peak compressed air system optimization requires addressing both the supply and demand sides of the system and understanding how the two interact.
Properly managing a compressed air system can not only save electricity, but also decrease downtime, increase productivity, reduce maintenance, and improve product quality.
Optimal performance can be ensured by properly specifying and sizing equipment, operating the system at the lowest possible pressure, shutting down unnecessary equipment, and managing compressor controls and air storage. In addition, the repair of chronic air leaks will further reduce costs.
For a typical compressed air end use, like an air motor or diaphragm pump, it takes about 10 units of electrical energy input to the compressor to produce about one unit of actual mechanical output to the work.
For this reason other methods of power output, such as direct drive electrical motors, should be considered first before using compressed air powered equipment. If compressed air is used for an application, the amount of air used should be the minimum quantity and pressure necessary, and should only be used for the shortest possible duration. Compressed air use should also be constantly monitored and reevaluated.
Air Compressor Types and Controls
There are two basic types of air compressors:
- Positive displacement, and
- Dynamic.
Positive Displacement.
In the positive displacement type, a specified quantity of air is trapped in a compression chamber and the volume which it occupies is mechanically reduced, causing a corresponding rise in pressure prior to discharge. Rotary screw, vane and reciprocating air compressors are the three most common types of air positive displacement compressors found in small and medium sized industries.
Dynamic.
Dynamic air compressors include centrifugal and axial machines, and are used in very large manufacturing facilities. These units are beyond the scope of this document.
Rotary Screw Compressors
Rotary screw compressors have gained popularity and market share (compared to reciprocating compressors) since the 1980s. These units are most commonly used in sizes ranging from about 5 to 900 HP. The most common type of rotary compressor is the helical twin, screw compressor. Two mated rotors mesh together, trapping air, and reducing the volume of the air along the rotors. Depending on the air purity requirements, rotary screw compressors are available as lubricated or dry (oil free) types.
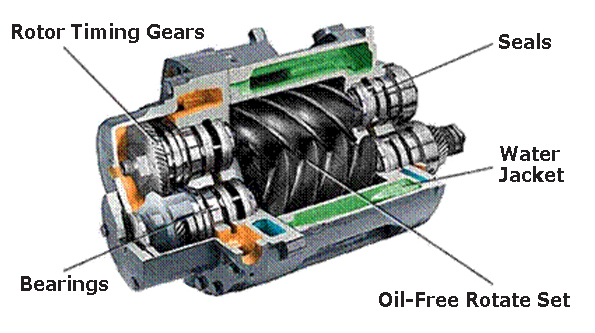
Text version
Figure 6 - Cross Section of a Representative Rotary Screw Compressor
cut-away illustration showing rotor timing gears, seals, water jacket, oil-free rotate set, and bearings.
Figure 6 - Cross Section of a Representative Rotary Screw Compressor
The biggest advantage of screw compressors over small air cooled reciprocating units is that they can run at full load continuously where the reciprocating compressors must be used at 60% duty cycle or below. Rotary screws are also a lot quieter and produce cooler air that is easier to dry. Be aware that rotary screw compressors may not be the most efficient choice compared to start/stop reciprocating compressors. Please refer to Case 3: On/Off vs. Load/No Load Control on page 101 for an example.
Lubricant Injected Rotary Screw.
The lubricant injected rotary screw compressor is the dominant type of industrial compressor for a perse set of applications. For lubricant injected rotary screw compressors, lubricants may be a hydrocarbon composition or a synthetic product. Typically a mixture of compressed air and injected lubricant exits the air end and is passed to a sump where the lubricant is removed from the compressed air. Directional and speed changes are used to separate most of the liquid. The remaining aerosols in the compressed air then are separated by means of a separator element within the sump, resulting in a few parts per million (ppm) of lubricant carryover in the compressed air. With two stage compressors, interstage cooling and the reduced internal losses due to a lower pressure across each stage increase the compression efficiency. Consequently, less energy is required to compress the air to the final pressure.
Dry Type Rotary Screw.
In the dry type, the intermeshing rotors do not contact one another, and their relative clearances are maintained to very close tolerances by means of external lubricated timing gears. Most designs use two stages of compression with an intercooler and aftercooler. Lubricant free rotary screw compressors have a range from 25 to 1,200 HP or 90 to 5,200 cfm.
Reciprocating Compressors
Reciprocating compressors have a piston that is driven through a crankshaft and by an electric motor. Reciprocating compressors for general purpose use are commercially obtainable in sizes from less than 1 HP to about 30 HP. Reciprocating compressors are often used to supply air to building control and automation systems.
Large reciprocating compressors still exist in industry, but they are now no longer commercially available, except for use in specialized processes such as high pressure applications.
Vane Compressors
A rotary vane compressor uses an elliptical slotted rotor situated within a cylinder. The rotor has slots along its length, each slot contains a vane. The vanes are forced outwards by centrifugal force when the compressor is rotating, and the vanes move in and out of the slot because the rotor is eccentric to the casing. The vanes sweep the cylinder, sucking air in on one side and ejecting it on the other. In general, vane compressors are used for smaller applications where floor space is an issue; however, they are not as efficient as rotary screw compressors.
Compressor Motors
Electric motors are widely used to provide the power to drive compressors. As a prime mover, the motor needs to supply sufficient power to start the compressor, accelerate it to full speed, and keep the unit operating under various design conditions. Most air compressors use standard, three phase induction motors.
For new or replacement air compressors, premium high efficiency motor should be specified over a standard ones. The incremental cost of the premium high efficiency motor is usually recovered quickly from the consequential energy savings.
For additional information about energy efficient motors, please refer to the Electric Motors Energy Efficiency Reference Guide published by CEATI.
Compressor Controls and System Performance
As air systems seldom operate at full load all of the time, the ability to efficiently control flow at part loads is essential.
Consideration should be placed to both compressor AND system control selection as they are important factors affecting system performance and energy efficiency.
Various inpidual compressor control strategies exist including the following:
- Start/Stop. This is the simplest and most efficient control strategy. It can be applied to either reciprocating or rotary screw compressors. Essentially, the motor driving the compressor is turned on or off in response to the discharge pressure of the machine. For this strategy, a pressure switch provides the motor start/stop signal. Start/Stop strategies are generally appropriate for compressors smaller than 30 horsepower in size.
Repeated starts may cause the motor to overheat and place greater maintenance demands on compressor components. For this reason, care should be taken in sizing storage receivers and maintaining wide working pressure bands to keep motor starts within allowable limits.
- Load/Unload. This control mode is sometimes called online/offline control. It keeps the motor running continuously, but unloads the compressor when the discharge pressure is adequate. Unloaded rotary screw compressors typically consume 15-35% of their full load power demand, while producing no useful compressed air output. Optional unload timers are available that will save energy by automatically turning off the compressor and placing it in standby if the unit runs unloaded for a period of time (usually 15 minutes).
Load/unload control strategies require significant control storage receiver capacity for efficient part load operation.
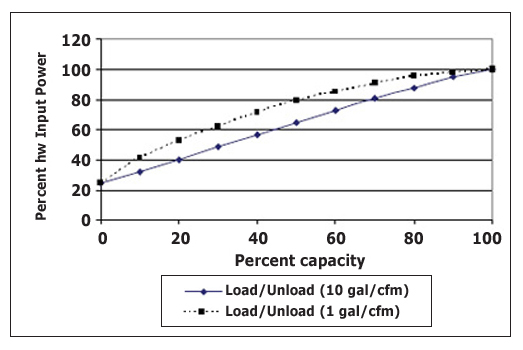
Text version
Percent kw Input Power | Percent capacity (Load/unload 1 gal/cfm) | Percent capacity (Load/unload 10 gal/cfm) |
---|---|---|
0% | 25% | 25% |
20% | 55% | 40% |
40% | 70% | 58% |
60% | 85% | 75% |
80% | 95% | 90% |
100% | 100% | 100% |
Figure 7 - Average Power vs. Capacity for Rotary Screw Compressor
- Modulating Control. This mode of control varies the compressor output to meet flow requirements by adjusting the inlet valve, resulting in air restrictions to the compressor. Even fully modulated at zero flow rotary screw compressors typically consume about 70% of their full load power demand. The use of pressure switch activated unloading controls can reduce the unloaded power consumption to 15 to 35%. Modulating control is unique to lubricated screw compressors and is the least efficient way to operate these units.
Compressor controls have a significant impact on energy consumption, especially at lower flows, where start/stop controls are generally the most energy efficient.
Figure 8 illustrates a typical performance curves for compressors where inlet valve modulation is used with and without unloading the compressor.
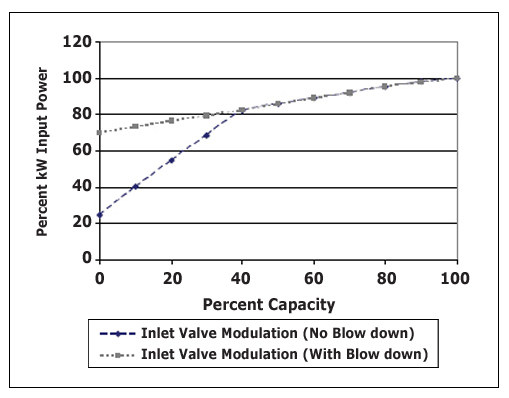
Text version
Percent kw Input Power | Percent capacity (Inlet valve modulation - no blow down) | Percent capacity (Inlet valve modulation - with blow down) |
---|---|---|
0% | 25% | 70% |
20% | 55% | 75% |
40% | 82% | 82% |
60% | 90% | 90% |
80% | 95% | 95% |
100% | 100% | 100% |
Figure 8 - Rotary Screw Compressor with Inlet Modulation Control
- Variable Displacement.
Some lubricated rotary screw compressors vary their output capacity using special capacity control valves, also called spiral, turn or poppet valves. With a variable displacement control scheme, the output pressure and compressor power consumption can be closely controlled without having to start/stop or load/unload the compressor. This control method has good efficiency at points above 60% loading. Use of pressure switch activated unloading controls at flows below 40% capacity can significantly reduce power consumption at lower flows.
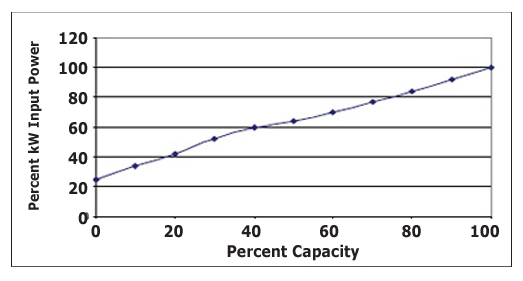
Text version
Percent kw Input Power | Percent capacity |
---|---|
0% | 25% |
20% | 40% |
40% | 60% |
60% | 70% |
80% | 80% |
100% | 100% |
Figure 9 - Rotary Screw Compressor with Variable Displacement
(Courtesy Compressed Air Challenge)
- Variable Speed Drive (VSD).
This control method varies the speed of the compressor to respond to changes in air demand. Both lubricated and oil free screw compressors can be purchased equipped with variable speed drive controls that continuously adjust the drive motor speed to match the variable demand requirements and maintain constant pressure. These compressors usually operate in on/off or load/unload control when air loading drops below the minimum speed of the drive.
In most cases a variable speed drive compressors offers the most efficient part load operation. Ideally, when there are multiple air compressors at a facility. One or more fixed speed compressors would supply the base load compressed air, and a VSD compressor would be used to supply the fluctuating or trim load.
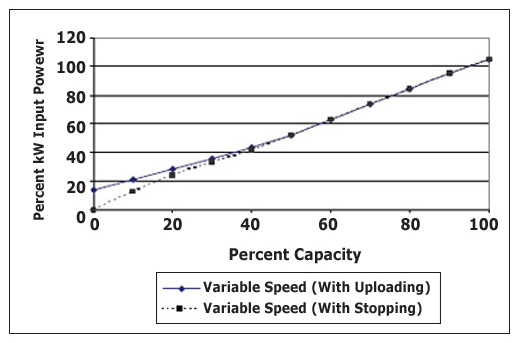
Text version
Percent kw Input Power | Percent capacity (variable speed - with uploading) | Percent capacity (variable speed - with stopping) |
---|---|---|
0% | 15% | 0% |
20% | 30% | 25% |
40% | 42% | 42% |
60% | 60% | 60% |
80% | 85% | 85% |
100% | 105% | 105% |
Figure 10 - Variable Speed Rotary Screw Power Curve
To benefit from VSD compressors, the appropriate amount of air receiver storage volume needs to be evaluated for different flow and control scenarios.
Variable speed drive (VSD) compressors should be considered for trim (or swing) duty as they are typically the most efficient unit to supply partial loads. Capable of supplying a constant pressure through a wide control range, the energy consumption and flow of a VSD compressor is almost directly proportional to the speed. This can result in energy savings over comparable fixed speed units when compressors are partially loaded. Be aware, however, that at full loads, the VSD will use slightly more energy compared to a similar sized constant speed motor drive.
Operating Cost Comparison of Different Control Modes
The compressor control mode can have a big effect on operating costs. In modulating mode the compressor would use 90% of full load power. For load/unload with minimal air storage (1 US Gal per cfm), the compressor would use about 92% of full power. By increasing the air storage to 10 US Gal per cfm, the load/unload compressor will use about 77% of full power. With variable speed drive control, the same size compressor will use about 66% of full power.
% Load | Modulating | Load/Unload with 1 gal/cfm Receiver |
Load/Unload with 10 gal/cfm Receiver |
Variable Speed Drive |
---|---|---|---|---|
100 | $36,130 | $36,130 | $36,130 | $36,850 |
75 | $33,420 | $34,680 | $29,350 | $27,090 |
65 | $32,330 | $33,240 | $27,820 | $23,480 |
50 | $30,710 | $31,070 | $24,200 | $18,060 |
25 | $28,000 | $24,930 | $16,800 | $9,030 |
10 | $26,370 | $16,620 | $11,740 | $3,610 |
*Based on 10 cents per kWh and 4,250 hours per year.
Multiple Compressor System Controls
The goal in controlling multiple compressors is to automatically maintain the lowest and most constant pressure, through all flow conditions, while ensuring all running compressors except one are either running at full load or off. The remaining compressor (trim unit) should be the one most capable of running efficiently at partial loads.
Local compressor controls independently balance the compressor output with the system demand and are always included in the compressor package. To achieve the stated goals, systems with multiple compressors require more advanced controls or control strategies (cascaded pressure bands, network or system master controls) to coordinate compressor operation and air delivery to the system.
Proper coordination is required to maintain adequate system pressures and increased efficiency whenever more than one compressor is required to run in a compressed air system.
Because compressor systems are generally sized to meet a facility’s maximum demand, but are normally running at partial loads, a method of control is required to ensure the running compressors are at their maximum efficiency. A description of some common control methods follows:
- Cascaded Pressure Band Control. This type of control is the simplest method of coordinating multiple compressors. With this control strategy the local compressor pressure switch controls are arranged in an overlapping or cascaded pattern (see Figure 12). This method of control will unload and/or load compressors at varying system pressures as the load decreases or increases. The cascaded control method results in higher than necessary system pressures during partial loads which causes higher than required energy consumption. Also, as the number of coordinated compressors increases, it becomes more and more difficult to achieve accurate compressor control without exceeding the pressure rating of the connected compressors at low loads or experiencing low system pressure at high loads.
Text version
Figure 12 - Multiple Compressor Cascading Control;
compressor #1 operates with a system pressure (psig) of 100 to 110 psig;
compressor #2 operates with a system pressure (psig) of 95 to 105 psig;
compressor #1 operates with a system pressure (psig) of 90 to 100 psig;
compressor #1 operates with a system pressure (psig) of 85 to 95 psig;Figure 12 - Multiple Compressor Cascading Control
- Network Control. This type of control uses the optional feature of the local compressor control to communicate with other compressors to form a chain of communication that makes decisions to stop/start, load/unload, modulate, and vary speed. One compressor generally assumes the primary lead with the others being secondary to the instructions from this compressor. This type of control can accommodate many compressors while maintaining system pressure within a single lower pressure band for all flow conditions. Typically these types of controls can only interconnect compressors of the same manufacturer.
- System Master Controls. (Also called automatic sequencers). Similar to network controls these externally installed controls interface with the local compressor controller to ensure system pressure remains within a single more efficient lower pressure band. Most system master controls can accommodate different manufacturers and types of compressors in the same system. Some newer system master controls have many extra capabilities, including the ability to monitor and control important parameters in the system.
- Multiple Controls with Variable Speed Drives (VSD). One or more VSD compressor(s) can be incorporated in the compressor control strategies previously indicated. In doing so it is important to ensure that the variable capacity is equal to or larger than the largest fixed speed compressor or a control gap will result. A control gap is where, under certain conditions, neither the base capacity nor the VSD will satisfy system loading. This control gap will cause the base and VSD compressors to fight for lead position and will lower system efficiency. Your compressor provider can assist you with properly sizing your VSD compressors.
To benefit from multiple compressor control, the appropriate amount of air receiver storage volume needs to be installed to slow system pressure changes and allow time to start and stop compressors. Storage is most important for load/unload control but is also required for systems using VSD compressors.
Compressed Air Auxiliary Equipment
Compressed air auxiliary equipment includes compressor aftercoolers, filters, separators, dryers, heat recovery equipment, lubricators, pressure regulators, air receivers, condensate drains, and automatic drains. They are devices associated with the air compressor and help to condition compressed air to the required specifications.
Air Compressor Coolers
Approximately 80 percent of the electrical energy going to a compressor is converted into heat. As an energy efficiency option, this heat of compression can be recovered and used to produce hot water or hot air. Compressed air heat recovery provides an excellent opportunity for energy efficiency at many facilities.
Compressor Cooling. Air compressors that operate continuously generate substantial amounts of heat from the heat of compression. This heat needs to be removed both from the air aftercooler and from the oil cooler. Compressor units are generally cooled with air or water.
- Intercoolers. Many older multiple stage compressors have intercoolers to remove the heat of compression between the stages of compression. These intercoolers should be cleaned periodically to maximize the heat transfer capability for energy efficiency.
- Aftercoolers. These units remove the heat of compression from both the compressor lubricant and the discharge air. The air cooler can be either air or water cooled and is installed after the last stage of compression. The proper operation of the air cooler is important because the moisture content of the air directly relates to discharge temperature. These coolers should be cleaned periodically to maximize the heat transfer capability for energy efficiency. Temperatures in excess of 38°C [100°F] will generally overload air dryers and cause moisture problems. Coolers reduce the temperature of the saturated discharge air and condense the water vapor, which must be separated and drained from the system. Maintenance of this drain is important to prevent free water from entering downstream drying equipment. Almost all industrial compressed air systems have some form of aftercooler.
- Compressor Intake. Although there is some debate in the industry, it is generally believed that air entering the air compressor should be as cool as possible for maximum energy efficiency. This is because cold air is denser than warm air. The colder the incoming air, the more the air molecules there are, so that more air is compressed for each revolution of the air compressor. Also the cooler the incoming air, the lesser the requirements for intercooling and aftercooling.
By ingesting an outdoor air intake supply (as opposed to air from a very warm compressor room), the energy efficiency is improved. When designing outdoor air intakes, pressure differential, freezing, and ice blockage in winter conditions need to be evaluated to maximize energy savings. Moreover, compressor rooms should be as clean and cool as possible to provide the foundation for optimal compressor operation.
Air Dryers
Compressed air leaving the compressor aftercooler and moisture separator is normally warmer than the ambient air and fully saturated with moisture. As the air cools the moisture will condense in the compressed air lines. Excessive entrained moisture can result in undesired effects like pipe corrosion and contamination at point of end use. For this reason some sort of air dryer is normally required.
Different types of compressed air dryers have different operating characteristics and degrees of dew point suppression (dew point is the temperature where moisture condenses in air).
Some end use applications require very dry air, such as compressed air distribution systems where pipes are exposed to winter conditions. Drying the air to dew points below ambient conditions is necessary to prevent ice buildup.
The typical pressure drop across a compressed air dryer is 3 to 5 psi. Some dryers found in industry are undersized and cause even higher pressure drops. For ongoing energy efficiency, compressed air should be dried only to the dew point required, and with the appropriately sized dryer.
Refrigerant, Regenerative and Membrane are the three main classes of air dryers.
Refrigerant Dryers. The main types of refrigerant dryers include:
- Non-cycling Refrigerant. (Also called direct expansion). This is the most common type of dryer as it has a relatively low initial cost. This type of dryer is appropriate for systems that can operate at dew points of greater than 2°C. The air dryer lowers the dew point of the air to the approximate temperature of the air exiting the refrigerant evaporator. To prevent freezing within the dryer, the evaporator temperature should not go below 0°C. Allowing for separator efficiency, air pressure dew point of 2°C, or higher for air leaving the dryer, can usually be obtained.
After first passing through a heat exchanger that transfers heat from the incoming air to the cooler outgoing stream, the dryer lowers the dew point of the air to the approximate temperature of the air exiting the refrigerant evaporator. This condenses the water vapor which is then removed in the dryer separator. To prevent freezing within the dryer, the evaporator temperature is usually regulated by bypassing the refrigerant past the evaporator using a hot gas bypass valve. This bypass keeps the refrigerant compressor loaded which causes the dryer to consume near full power even when lightly loaded, resulting in poor part load efficiency.
Energy can be saved by turning off the air dryer during the hours when the air compressor is off.
- Cycling Refrigerant. (Also called thermal mass). These dryers have the same dew point rating as non-cycling dryers but, rather than using a hot gas bypass valve to control evaporator temperature, the dryer uses a thermal mass to store cooling. This allows the refrigerant compressor to operate in on/off mode without over-cycling. The cycling dryer has a very good turn-down in response to both reductions in flow and moisture loading, resulting in good part load efficiency.
- Variable Speed Refrigerant. This dryer type uses electronic means to match the drying capacity with system demand. This style of dryer has good part load efficiency.
Regenerative Dryers. These desiccant dryers use a porous material to dry the air. Once the desiccant material becomes saturated, it must be regenerated. Various types of regenerative dryers use different methods of regeneration. These dryers are capable of removing moisture to levels found well below the freezing point of water (e.g. -40°F or °C or below). However, the purge air requirement to regenerate the dryer can impose a major energy penalty to the system.
Dewpoint controls are available that can match the regeneration energy requirement to air system demand. Dewpoint controllers should be used for energy efficiency improvements.
The main classes of Regenerative Dryers include:
- Heatless Regenerative. This type of dryer uses already dried compressed air to regenerate the desiccant. An uncontrolled heatless air dryer will constantly use 15 to 20% of its nameplate rating to perform this operation. This flow costs about 3 to 4 kW per 100 cfm of dryer nameplate rating.
- Exhaust Purge Regenerative. This type of dryer takes a smaller amount (7 %) of already dried air from the system and passes it though an electric heater. This heated air is more effective in stripping the moisture from the dryer desiccant. This type of dryer costs about 2.25 kW per 100 cfm of dryer nameplate rating to operate.
- Blower Purge Regenerative. This dryer uses a blower to pass heated ambient air through the desiccant for regeneration. During this operation no compressed air is used which means the full compressor output is available to the system. A flow of compressed air is usually used for cooling after the heating cycle. These units consume about 2.5 kW per 100 cfm nameplate rating. Cooling purge, if used, consumes another 0.6 kW per 100 cfm.
Membrane Dryers. These units use a semi-permeable membrane to separate water vapour from the air stream. They have no moving parts. The units use about 20% or the nameplate rating to sweep the membrane. This sweep air is lost to the air system. These dryers exhibit variable dew point output depending on the flow of air and the temperature.
% Load | Non-cycle Refrigerant |
Cycling Refrigerant |
Heatless Regenerative |
Heatless Control Regenerative |
Membrane Dryer |
---|---|---|---|---|---|
100% | $1,070 | $1,070 | $6,390 | $6,390 | $8,520 |
75% | $1,070 | $800 | $6,390 | $4,790 | $8,520 |
50% | $1,070 | $530 | $6,390 | $3,200 | $8,520 |
25% | $1,070 | $270 | $6,390 | $1,600 | $8,520 |
10% | $1,070 | $110 | $6,390 | $640 | $8,520 |
0 | $1,070 | 0 | $6,390 | 0 | $8,520 |
*Based on 500 cfm dryer capacity running 4,250 hours per year at 100 psig and 10 cents/kWh.
Figure 13 - Operating Costs of Different Dryer Types*
After factoring energy and capital equipment costs, remember that it costs 5-10 times as much to dry the air to -40°C as compared to drying it to +2°C.
Filters
Compressor Air Inlet Filter. An air inlet filter protects the compressor from atmospheric airborne particles, insects and plant material.
Inlet filters should be replaced periodically, especially in areas prone to dust and insects. High inlet filter pressure differential reduces the output capacity of an air compressor and decreases its efficiency.
Compressed Air Filters. Compressed air filters downstream of the air compressor are generally required to remove contaminants, such as particulates, condensate, and lubricant. Numerous choices for filtering exist depending on the cleanliness of the air required.
Generally the finer the filter, the more pressure differential exists across the element. Particulate filters are used to remove solid particles and have the lowest differential.
Coalescing filters that are used to remove lubricant and moisture usually have the highest differential. Particulate filters are generally used just after a desiccant dryer to remove desiccant fines. A given filter pressure differential increases to the square of the increase in flow though it. This filter differential increases the compressor energy required to produce a fixed downstream pressure.
About 1% in higher energy costs results from every 2 psi in filter differential. If a given filter capacity is doubled the pressure loss across it will reduce by a factor of 4, for a 75% savings. From an energy efficiency perspective, air filter types should be chosen carefully as there is an energy penalty for over filtering.
For oil and particulate filters, use filtration only to the level required by each application. Filter differential should be carefully monitored and filter elements replaced in accordance with manufacturers' specifications or when pressure differential causes excessive energy consumption. Accurate pressure differential gauges should be used to monitor pressure differential.
To save energy, where possible, minimize the filter pressure drop by using low differential mist eliminator style filters, oversized filters, or by using filters installed in parallel.
Be aware that excessive filter differential often causes higher compressor energy consumption due to short cycling of the compressor.
Example 1 - Cost of Clogged Filters
Consider a 100 HP compressor operating for 2 shifts with a required discharge pressure of 100 psig. The annual electricity cost of this compressor is $32,330 (see Figure 4). If this unit instead had to operate at 110 psi to overcome a total filter differential of 10 psi, the energy penalty would be approximately 5% or $1,615 per year. In most cases proper design can reduce pressure differential to less than one psi.
Receivers and Air Storage
The presence of adequate storage receiver capacity helps to maintain air quality, air system stability and air system efficiency. Adequate air storage is extremely important in systems using screw compressors.
Receivers can be Primary or Secondary as discussed below.
Primary Air Receivers
A primary air receiver acts as general system storage and is usually located close to the main air compressors and can be located upstream and/or downstream of the clean-up equipment.
Primary air receivers have a number of important uses in air systems:
- Damping pulsations caused by reciprocating compressors.
- Providing a location for free water and lubricant to settle from the compressed air stream.
- Supplying peak demands from stored air without needing to run an extra compressor.
- Reducing load/unload or start/stop cycle frequencies to help screw compressors run more efficiently and reduce motor starts. Most screw compressors have internal protection that prevents more than 4 to 6 starts per hour.
- Slowing system pressure changes to allow better compressor control and more stable system pressures.
As a rule of thumb, for load/unload operated lubricated screw compressors, the receiver volume should be 5 to 10 US gallons (20-40 liters) per trim compressor scfm output. Other factors come into play when sizing, such as the type of air compressor method of capacity control and compressor starting delays.
The location of the primary receiver can have a significant effect on the air dryer. Receivers located downstream of the air dryer can store large quantities of dry air for use in feeding peak demands. If there is a sudden demand in excess of the compressor capacity the stored air can flow directly from the receiver to help maintain adequate pressure. If, on the other hand, the primary receiver is located on the upstream side of the dryer the combined flow from the compressor and the receiver must flow through the dryer. This can cause flows that exceed the dryer capacity. For this reason the largest primary receiver should be located downstream of the dryer and filters.
Secondary Air Receivers
Secondary receivers (located in the distribution system of a facility or at and end use) have the following general uses:
- Protection of sensitive end uses from temporary system pressure dips.
- Protection of multiple end uses from large transient users of compressed air.
- To provide general pressure stability in systems with undersized distribution piping.
Many industrial plants have equipment located at the end of a long air distribution pipe, or machinery requiring large amounts of compressed air for short periods of time. This condition often results in severe localized pressure fluctuations with many essential end points being starved for compressed air. Sometimes this situation can be relieved by correctly sizing and locating a secondary air receiver close to the point of high intermittent demand. If the intermittent demand occurs over a short duration, it may be possible to supply the required air directly from the storage tank rather than running added compressor capacity. By installing a flow restriction before the secondary air receiver, the storage tank can be refilled at a reasonable lower flow rate so as not to affect other local pressure sensitive end uses.
Alternatively, if a single low-flow pressure sensitive end use is being affected by local pressure fluctuations, a properly sized storage receiver with a check valve can be installed that will trap compressed air for exclusive end use. In this way a sensitive device can ride through the occasional pressure fluctuation.
Generally a receiver of about 110 US gallons (415 l) will store 1 cubic foot of compressed air per psi. Required receiver size for any application is simply the cubic feet required multiplied by 110, and then divided by the pressure range.
Example 2 - Determining Size of Air Receiver
A clamp using 2 cfm needs a check valve protected storage receiver to maintain at least 85 psi for 2 minutes in a system that normally operates at 100 psi.
- Cubic feet required = 2 cfm x 2 minutes = 4 cubic feet
- Pressure (psi) range during even = 100 - 85 = 15
- Storage receiver required 4 x 110/15 = 29 gallons (US)
Facilities having large fluctuations in air demand, or having insufficient air pressure (usually at the end of the line), should evaluate the need for one or more air receivers strategically located in the air distribution system.
Separators and Drains
Water separators are devices that remove entrained liquids from the air. They are installed following aftercoolers to remove the condensed moisture. Water separators should not be confused with oil separators which are used within lubricated rotary screw compressors to recover lubricant from the compressed air discharge.
Drains are needed at all separators, filters, dryers and receivers in order to remove the liquid condensate from the compressed air system. Failed drains can allow slugs of moisture to flow downstream that can overload the air dryer and foul end use equipment. Poorly designed or maintained drains tend to waste significant compressed air.
Replacing a manual drain constantly consuming 5 cfm of air in a two shift (4,250 hours per year) operation would save about $425 per year.
There are four main methods to drain condensate:
- Zero air loss traps with reservoirs. Most efficient design as only condensate is expelled. Normally easy to test and maintain. Because the condensate is normally gravity fed the installation configuration is critical to prevent air lock.
- Electrically operated solenoid valves. If set to drain worst case moisture loading this drain style will waste air during periods of lower moisture demand. The solenoid operated drain valve opens for a specified time based on a preset adjustable interval. In some instances, the time the valve is open is not sufficient for adequate condensate drainage.
- Float operated mechanical drains. Normally difficult to test to determine if working and difficult to maintain. Often points of leakage. These traps do not waste air when operating properly, but they often require significant maintenance as they are prone to blockage from sediment.
- Manual drains. Manual valves used to discharge condensate are often located at points where moisture problems are experienced. As these valves are not automatic, in many instances, manual valves are left partially cracked open allowing compressed air to constantly escape. This type of drainage should be avoided.
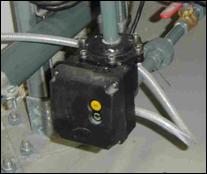
Figure 14 - Example of Zero Air Loss Drain
Piping
Piping delivers compressed air from the compressor room to end use equipment and processes. Most compressed air systems use carbon steel piping, which is subject to corrosion. Hence many compressed air systems require an air dryer to control the moisture level. An undersized piping system in the compressor room or distribution line will cause a significant pressure loss between the compressed air production system and the end uses. To be able to maintain a given fixed downstream pressure this pressure differential causes an increase in compressor discharge pressure which increases the compressor energy requirement by about 1% for every 2 psi increase.
Points to consider when dealing with piping:
- Horizontal lengths of distribution piping should be sloped slightly downwards, with provision for moisture drainage.
- When designing a compressed air system, it is often a good practice to add 30% to the expected air flow (to add for future potential system expansion), and then select the pipe diameter having the lowest pressure drop.
- If possible it is good practice to loop the distribution piping in order to allow for air to travel in multiple directions, as illustrated in Figure 17. A single loop of pipe can reduce pressure differential by 75% compared to a single pipe of similar size. Multiple loops can further enhance the flow of air.
To minimize energy loss from pressure differential and to help stabilize the end of line air pressures, the distribution system should be sized for no more than 2-3 psi pressure differential.
- Consider choosing a piping material with a lower coefficient of friction such as copper or extruded aluminum for lower pressure loss.
- Consider using larger diameter pipes to take advantage of lower pressure differential.
If a pipe area is doubled, for a given flow, there is generally one quarter the pressure drop for the single diameter pipe - a 75% energy savings.
Figure 15 shows the relationship between pressure drop per 1,000 feet (304 m) of pipe length, in various pipe diameters for a given air flow rate; however, any pressure drop greater than 40 psi is unrealistic in practice.
Caution: The table in Figure 15 is an indicator only and should not be used for design purposes.
Cubic Feet of Air (scfm) |
Pipe Diameter in Inches | ||||||
---|---|---|---|---|---|---|---|
1" | 2" | 3" | 4" | 6" | 8" | 10" | |
10 |
0.28 |
||||||
50 |
9.96 |
0.19 |
|||||
100 |
27.9 |
0.77 |
|||||
250 |
4.78 |
0.58 |
|||||
500 |
19.2 |
2.34 |
0.55 |
||||
750 |
43.3 |
5.23 |
1.24 |
||||
1,000 |
76.9 |
9.3 |
2.21 |
||||
1,500 |
21.0 |
4.9 |
0.56 |
||||
2,000 |
37.4 |
8.8 |
0.99 |
||||
2,500 |
13.8 |
1.57 |
0.37 |
||||
3,000 |
20.0 |
2.26 |
0.53 |
||||
4,000 |
35.5 |
4.01 |
0.94 |
0.28 |
|||
5,000 |
55.6 |
6.3 |
1.47 |
0.44 |
Pipe Diameter (inches) | Equivalent Flow (scfm) |
---|---|
1 | 36 |
2 | 263 |
3 | 431 |
4 | 909 |
6 | 2,679 |
8 | 6,757 |
10 | 14,286 |
For every 2 psi pressure drop caused by undersized or bottlenecked piping, there is approximately 1% increased energy required.
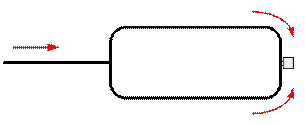
Figure 17 - Looped Piping Layout - Multiple Paths for Air Supply
In order to minimize pressure drops, where possible, elbow and angle joints should be smooth and gradual. This may not be possible with threaded black iron fittings but often with careful planning the system can be laid out in a way that would minimize direction changes.
Joints, bends and connection points to distribution pipes cause pressure drops. Figure 18 gives and indication of the approximate pressure drop for selected fittings in terms of equivalent length of straight pipe.
Caution: The table in Figure 18 is an indicator only and should not be used for piping system design purposes.
Friction Loss Equivalent Length - feet of Straight Pipe (ft) | ||||||||
---|---|---|---|---|---|---|---|---|
Fitting | Nominal Pipe Size (inches) | |||||||
0.50 | 0.75 | 1.00 | 1.50 | 2.00 | 3.00 | 4.00 | 6.00 | |
90° Elbow | 1.5 | 2 | 2.5 | 4 | 5.7 | 7.9 | 12 | 18 |
45° Elbow | 0.8 | 1.1 | 1.4 | 2.1 | 2.6 | 4 | 5.1 | 8 |
Gate valve | 0.3 | 0.4 | 0.6 | 1 | 1.5 | 3 | 4.5 | 6.5 |
Tee Flow - Run | 1 | 1.4 | 1.7 | 2.7 | 4.3 | 6.2 | 8.3 | 12.5 |
Tee Flow - Branch | 4 | 5 | 6 | 8 | 12 | 16 | 22 | 32.7 |
Male /Female Adapter | 1 | 1.5 | 2 | 3.5 | 4.5 | 6.5 | 9 | 14 |
Flow Controllers
These devices (sometimes called intermediate controllers or expanders) are typically located near the air compressor room discharge. They stabilize system pressure with more precision than compressor controls. These units can be pneumatically controlled or have very accurate electronic PID control. Much better air system pressure stability and a more efficient lower average facility pressure can be achieved using these valves.
For unregulated air demands, the higher the average pressure the more compressed air used by plant air leaks and end uses. This additional flow is called artificial demand. This demand causes higher compressor energy consumption.
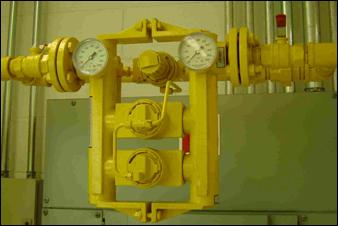
Figure 19 - Flow Controller
Savings of about 1% per psi reduction can be gained due to lower pressure if a significant portion of the load is unregulated. This savings effect is minimal if the majority of the end uses are regulated.
Some items to consider when using flow controls are:
- Flow controllers isolate the compressors from downstream storage receivers. It is important to have significant storage on the compressor side of the flow control or excessive compressor cycling and higher compressor energy consumption will result.
- There is an energy penalty in running the compressor discharge pressure at a higher than normal level on the upstream side for the flow control. Operating at higher pressure, however, means more air can be stored which can prevent the start of an addition compressor during peak loads and avoiding peak demand penalties. The energy cost of doing this should be carefully considered.
Filter Regulator Lubricator Devices
Filter Regulator Lubricator. The pressure regulation for air systems can be located at the end use. In many cases the regulator is part of an assembly called a filter, regulator, lubricator (FRL). A lubricator may be situated near a point of end use to lubricate pneumatic tools and other machinery. The lubricator is sometimes combined with a filter and a pressure regulator in the form of a FRL.
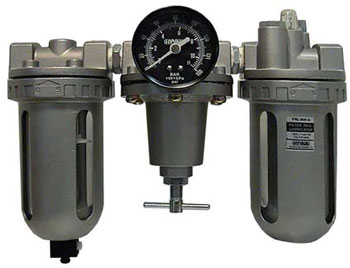
Figure 20 - Filter Regulator Lubricator (Courtesy Hyvair)
When dealing with FRLs, the following points should be considered:
- The filter selected should be sized for minimal pressure differential at the highest flow connected to the assembly. Consult manufacturers' curves which are available in most equipment data sheets.
- Precision regulators with large diaphragms should be used to reduce the typical pressure droop that is characteristic of low cost regulators. Consult manufacturers' curves to determine the pressure characteristics.
- Regulators that are cranked wide open (set to track system pressure) cause a pressure differential during peak flows. Consider removing these units for better end use pressures.
- Lubricators should be kept filled to ensure proper lubrication of the connected tools. A properly lubricated air tool works better and uses less air.
Fittings
Fittings and couplings for air hoses need to be durable and airtight. Many clamps used for air hoses are purchased for lowest cost, and are subject to poor performance after a given period of time as highlighted in Figure 21.
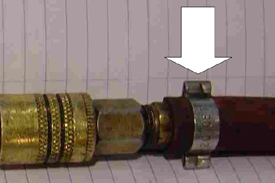
Figure 21 - Example of Hose Clamp Subject to Leaks
Quick coupling fittings can be a major point of pressure drop. Often the air must flow through more than one coupling to feed the end use, with each coupling causing up to 7 psi pressure differential at its rated flow. It is not uncommon to see pressure differentials of up to 30 psi across the hoses and couplings. This can be addressed by upsizing connectors and hoses for minimal pressure differential.
Hoses may be retrofitted with sturdier crimping clamps and quick connect/disconnect air tight fittings as illustrated in Figure 22.
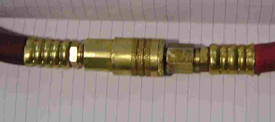
Figure 22 - Air Tight Air Hose
Uses and Misuses of Compressed Air
Compressed air is used for a diverse set of applications in industry (See Figure 23). The cost to produce compressed air is often unknown to the users, and for this reason, it may be used in an inefficient manner.
For many applications, other sources of power may be more cost effective and energy efficient. Typically, less than 10% of the original energy used to produce compressed air is actually converted into useful work by the end use application. Think about the equipment at your facility and ask if any of the end uses can be converted to other power sources.
Figure 23 - Common Uses for Compressed Air
Agitating liquids | Cooling | Pressure treatment |
Air brakes | Dehydration | Sawing |
Air piston powering | Fertilizing | Seeding |
Blending | Forming | Snow making |
Bottling | Hoisting | Spraying coatings |
Clamping | Injection molding | Sprinkler systems |
Cleaning | Mixing | Stamping |
Controls and actuators | Mold press powering | Tool powering |
Conveying | Packing | Vacuum melting |
Inappropriate Uses of Compressed Air
Inappropriate uses of compressed air are defined as applications that could be powered more efficiently or economically using an energy source other than compressed air.
Although one of the most expensive forms of plant energy, compressed air is easily accessible, and simple to adapt for use. Consequently it is commonly used for applications where other energy technologies and energy inputs would be more efficient and economical.
Examples of common potentially inappropriate uses of compressed air are shown in Figure 24.
Inappropriate Compressed Air Use | Description and Examples | Potential Solutions |
---|---|---|
Abandoned Equipment |
|
|
Aspirating |
|
|
Atomizing |
|
|
Dense phase transport |
|
|
Open blowing |
|
|
Equipment or Personnel cooling |
|
|
Unregulated Equipment |
|
|
Vacuum generation |
|
|
So You Want to Perform a Compressed Air System Assessment?
Evaluating your compressed air system is the first step in improving its energy efficiency performance. Facilities may undertake compressed air system assessments using in house expertise or, possibly, through a qualified consultant or contractor. The initial evaluation involves a one time concentrated effort supplemented by ongoing focused spot checks. It should take a systems approach - that is an examination of individual components, and how they interact.
A qualified practitioner can assist you in performing the assessment and making system improvement recommendations. Contact your utility for a list of qualified and experienced firms in your area. Many provincial and municipal electric distribution companies offer technical support for these assessments. Financial aid may be available from federal and provincial governments and local utilities to defray part of the cost of doing a system assessment and implementing capital improvements.
Once the important operating parameters of the complete system are measured, and the operation of the system well understood, the areas requiring attention will come into focus. Supplementary improvement efforts need to focus primarily on the deficiencies identified during the initial assessment, together with ongoing monitoring of air leaks, system pressures, flows, temperatures, air quality, energy consumption and system control set points. The common steps in establishing a compressed air system improvement program include:
- Gathering equipment nameplate data, receiver volumes, piping sizes and lengths and creating a drawing of the system.
- Establishing a baseline by measuring the current compressed air performance levels.
- Establishing required performance levels for system pressure, power consumption, and air quality.
- Analyzing performance data, reviewing operating history, gathering and calculating operating costs to identify areas that require improvement.
- Assessing alternative system configurations and other improvement measures to determine the best technical and economic options.
- Determining the best technical and economic options to optimize the sub-components.
- Devising a plan to implement the improvements for ongoing optimization.
- Examining maintenance and purchasing practices.
- Factoring in current deficiencies and future facility requirements for compressed air.
- Asking yourself if there is a better way to supply and use compressed air.
Compressed air needs are defined by the air quality, quantity, and level of pressure required by the end uses in your facility. Analyzing needs carefully will ensure that a compressed air system is configured properly. The higher the quality, the more the air costs to produce. Higher quality air usually requires supplementary equipment, which not only increases original capital investment, but also makes the overall system more expensive to operate in terms of energy consumption and maintenance costs.
Gathering Equipment Data
A first step in the process is gathering equipment data. This can be found by recording nameplate data, service records, operating manuals and purchase orders.
This inventory should include recording the nameplate information and setpoints for all of the equipment in the compressed air system including the air compressor(s), aftercoolers, air dryers, receivers, filters, and controllers. A sketch should be made of your compressed air production and distribution system layout noting the pipe sizes, air take off points, and valves. The type and characteristics of machinery or tools along the route of the compressed air system should be recorded.
Establishing a Baseline
Several parameters should be measured to determine the baseline performance of your system. The baseline should normally include a load profile. A compressed air load profile indicates how demand for air and compressor energy consumption changes over time. A facility with short periods of heavy demand may benefit from implementing storage options, whereas a facility with a varying load profile will likely benefit from advanced control strategies. An example of a load profile graph appears in Figure 25.
The following measurements, assessments and calculations are normally included in a system assessment:
- Air pressure measurements over time.
- System pressure differentials at various locations between the compressor discharge and the important end uses.
- Compressor Amps or kW vs. time. (Note: Only properly qualified personnel should undertake electrical measurements.)
- System flow (either calculated or directly measured) preferably over time. This can be easily calculated using loaded vs. total run time for compressors with hour meters.
- Ambient and compressed air temperatures.
- Calculated operating costs for electricity, (water or chilled water), maintenance and taxes based on the gathered data.
- System leak identification and measurement.
- End use equipment pressure drops or differentials.
- Identification of inappropriate uses of compressed air.
- Assessment of air filtration systems for pressure drops and effectiveness.
- Evaluation of air storage receivers.
- Assessment of air dryers (required dew points, energy consumption and pressure drops).
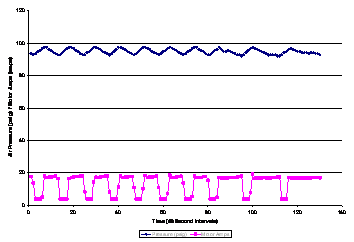
Figure 25 - Example of Compressed Air Pressure and Motor Amp Profile
(Courtesy Manitoba Hydro)
Analyzing Performance Data and Establishing Performance Levels
Once measurements are taken and performance standards established, the data can be analyzed to determine if the system is meeting the facility's needs. The analysis will point to areas of deficiency and identify potential opportunities for improvement.
Optimizing peak compressed air performance requires an examination of the relationship and interaction of components acting as a system, plus an independent component evaluation.
Areas to evaluate include:
- Compressor type, size and condition. The compressors are evaluated for appropriateness of the intended use as well as overall condition. Compressor efficiency can be estimated from manufacturer specifications that are corrected to site conditions. The compressor installation is also evaluated for location, air intake, ventilation, and heat recovery.
- Primary and Secondary Receivers. The effectiveness of the receiver tank should be evaluated for location and size. For the most part, the air compressors should be able to supply the plant's air needs, except for short periods of high demand that can be supplied by one or more receivers. Secondary air receivers to control demand events should also be investigated.
- Compressor Controls. Check for appropriate pressure set points. In the case of multiple compressors, the pressure bands to trigger the start or stop of a compressor need to be adjusted.
- Filters should be examined for cleanliness and appropriateness for the application. Pressure drops across the filters should be evaluated to estimate energy losses attributable to the filter. Check the appropriateness of maintenance schedules for changing the filters. Consider purchasing higher performance filters.
- Aftercooler and moisture separator efficiency and cooling effectiveness can be measured and feasible modifications or alternative systems recommended.
- Dryer appropriateness needs to be assessed based on the facility's end use for compressed air. It is important to note the dryer size, pressure drops, overall dryer efficiency, and consider dryer modifications based on the volume and quality of air requirements.
- Automatic Drains. The location, condition, and effectiveness of all drains needs to be evaluated and energy efficient alternatives recommended where appropriate.
Other areas to consider are:
- System pressure stability (is the plant having pressure problems)
- System specific power (how many kW does it take to produce 100 cfm) or how many dollars does it cost per 100 cfm
- Dewpoint stability (is there water in the air)
- Peak, minimum and average flows (can the production system to adequately supply these flows)
- Peak, minimum and average compressor room temperatures (can the compressors and dryers operate adequately in these conditions)
- Maintenance and operating costs per year and per hour of operation (is it costing more to maintain a compressor than to purchase a new unit)
Devising a Plan
Once peak and average flows are known and performance levels established it is possible to calculate energy savings numbers based on various alternatives.
Some things that could be considered include:
- Identification of equipment that can be shut down
- Selection and use of compressor and flow controllers
- Opportunities to downsize or purchase new equipment where appropriate
- Evaluation to minimize compressed air equipment operating hours
- Proper selection of air compressors (number of stages, type of air compressor, and control modes)
When selecting new or replacement equipment for your compressed air system, remember to base your decision on the overall expected life cycle operating cost. Avoid purchasing decisions made on initial price alone. Obtain Compressed Air Gas Institute (CAGI) compressor performance specifications to compare the full load performance of one brand versus another. In addition to providing you with unbiased advice, your local utility can often suggest the names of qualified practitioners in your area to help you with your compressed air plans.
Points to Consider When Hiring a Compressed Air Auditor
From time to time you may require help in undertaking a compressed air assessment or to plan a new system expansion. Here's a list of questions to think about in helping you make your decision¹:
- What's the track record and knowledge level of the firm and individual who will undertake the work?
- How well does the service provider understand energy efficiency and economic tradeoffs?
- How familiar is the service provider with all aspects and types of compressed air, including air supply, and air demand?
- How well do they know other associated equipment (dryers, filters, receivers, piping, end use etc.)?
- How well does the service provider understand my industry and the products we manufacture or process?
- How objective will the report or advice be? (e.g., Are they just trying to sell us more equipment or services, or is the work being done impartially and independently?)
- How responsive is the service provider? (availability to do the testing to minimize impacts to the facility and/or undertake the testing during nights/weekends)
- Can the testing be done with minimum supervision?
- What will the compressed air assessment include, and what will the final report look like?
- Can the final report be presented to management for developing an energy efficiency and business case?
- How responsive is the service provider to health and safety practices and procedures
Proven Energy Efficiency measures
This section describes time-proven measures to improve the energy efficiency of compressed air systems, including:
- Identifying and repairing air leaks
- Minimizing pressure drops
- Minimizing end use of compressed air
- Examining compressor heat recovery
- Optimization of air production equipment
Compressed Air System Leaks
Air leaks can be a significant contributor of wasted energy in a compressed air system, and in some instances lead to productivity losses. It is not unusual to encounter 20 to 30 percent of a compressor's output in the form of air leaks at typical industrial facilities. Proactive leak management programs (detection and repair) can reduce leaks to less than 10 percent of a plant's compressed air production.
Experience has shown, time after time, that fixing air leaks is most often the top priority for any compressed air system optimization. Typically you will find that your efforts will have a simple payback of less than 6 months.
In addition to being a source of wasted energy, leaks can also contribute to other operating losses. There is strong cause and effect relationship between the number and magnitude of air leaks with the overall compressed air system pressure. For example, lower air pressure can affect air tools and equipment by reducing the mechanical output and decreasing the resulting productivity of the process.
Indifference to air leak management can lead to purchasing unnecessary air compressor capacity, thereby increasing capital expenses.
Figure 26 shows the approximate annual cost for electricity ($0.10/kWh) for different size leaks based on one, two and three shift operation.
The type of compressor control can have a large effect on the results of any leak reduction effort. For example, a leak reduction effort that reduces the air consumption by 10%, in a system with a single modulating compressor, would only achieve about 3% in energy savings because of the limited turndown capability of the modulating compressor control. This same reduction when applied to a system with a VSD compressor would result in energy savings of about 10%.
Leak size | 1 Shift (2250 hrs) |
2 Shifts (4250 hrs) |
3 Shifts (8400 hrs) |
---|---|---|---|
1/16" leak | $200 | $380 | $750 |
1/4" leak | $3,210 | $6,070 | $11,990 |
3/8" leak | $7,230 | $13,650 | $26,980 |
1/2" leak | $12,820 | $24,210 | $47,850 |
Estimating Total Air Leaks
A good first step in addressing air leakage in a plant is to do a low load test during a non-production time. This might be fairly easy if there is an existing accurate flowmeter already installed in the system or if the air compressors have capacity gauges. If not, a special test can be performed using one or more plant air compressors.
If the plant compressors already operate in load/unload mode (a compressor service provider can assist in determining this) a leak estimate can be made by measuring the loaded and unloaded times while the compressor is feeding the leaks. For example if a 100 HP compressor rated at 400 cfm is loaded for 2 minutes and unloaded for 3 minutes, the leak load can be estimated by taking the loaded time and dividing the total loaded plus unloaded time, or for this example 2/5 = 0.4. This indicates the compressor is loaded 40% of the time. The leak load would then be 40% of 400 cfm or 160 cfm. If another compressor was loaded during this time its capacity would be added to this calculated value. Generally the output capacity of any compressor operating around 100 psi would be about 4 times the compressor nameplate horsepower rating.
This test can also be done with modulating compressors using an accurate pressure gauge and a stopwatch. This test causes wide pressure fluctuations so it is important to determine if critical equipment will be affected.
If the plant can be run on one compressor, test the leak load by turning the compressor off and measuring the time it takes for the pressure to drop from a point 10 psi lower than the normal system pressure to a point 30 psi lower (20 psi drop). The test is done at this lower point to prevent compressor modulation during the test.
For the second part of the test, turn on the compressor and measure the time it takes for the pressure to rise though the same two pressure points. Repeat the test a number of times, being careful not to exceed the 4 motor starts per hour. The compressor loaded ratio is determined by taking the rise time and dividing by the total time (rise plus fall). As in the previous example, the leak load is estimated by multiplying this ratio by the compressor cfm output. If a second compressor was required to get to the required pressure its capacity would be added to the total.
The approximate cost to feed these leaks at 100 psi can be determined as follows:
0.2 x leak cfm x hours per year x cost per kWh
A 100 cfm leak rate would cost about 0.2 x 100 x 4,250 x $0.10 = $8,500 per year to maintain at 10 cents per kWh blended rate.
How to Track Down Air Leaks
Air leaks are very difficult to see or hear in environments with high background noise (e.g., fans and machinery).
When the plant is shut down, you can often hear the air leaks. If background noise is present, you will probably need to use an ultrasonic leak detector. Ultrasonic acoustic detectors are handheld devices that recognize the presence of air leaks by their ultrasonic sound patterns. Once a general location of an air leak is determined, soapy water may be applied to suspected areas. The soapy water method is very reliable, however it is time consuming to undertake.
The best time to find air leaks is when the plant is not operating, usually at night or on weekends. Walk the length or perimeter of the compressed air distribution system. Stop every so often and listen for air leaks. Look for damaged fittings or cracked hoses. Write down and sketch the location of the air leaks. Use tags to mark the location of air leaks for repairs. Repeat the process periodically as part of your maintenance routine.
Caution: Always use appropriate vision and hearing protective equipment, and follow proper safety procedures when detecting air leaks or when working at elevated heights.
Experience has shown that air leaks occur most often at joints and connections. Fixing leaks can be as simple as tightening a connection or replacing root cause faulty equipment including:
- Couplings
- Fittings
- Pipe sections
- Hoses
- Joints
- Drain Traps
- Valve stems
Preventing Air Leaks
Here are some tips to help prevent leaks from happening in the first place:
- Install fittings properly with appropriate sealants where applicable.
- Isolate non-operating equipment with a valve in the distribution system.
- Lower the air pressure of the system where possible. A lower pressure differential across an air leak reduces the rate of flow by a small amount. This however is not a cure for fixing air leaks.
- Select high quality fittings from reputable suppliers including air hoses, tubing, disconnects.
Remember that once leaks have been repaired, the compressor control system often needs to be adjusted so as to achieve the true energy savings potential.
Lower Compressor Discharge Pressure by Minimizing Pressure Drops
Compressor discharge pressure affects the efficiency of an air compressor. In rare cases the compressor discharge pressure will have been inadvertently set too high for no valid reason. In these cases energy can be saved by simply readjusting the compressor control setpoints to a lower level. This should be done carefully and in small steps so as not to affect sensitive plant equipment.
Most often, however, compressor discharge pressure is set artificially high to overcome various system pressure drops between the compressor and critical end uses. Pressure drop is caused by restriction to flow that is internal to system pipe work and components. Too much pressure drop can result in poor system performance and excessive compressor energy consumption.
The higher the discharge pressure from the air compressor, the more money and electricity it costs to produce the compressed air. Often times, the air compressor's discharge pressure is set at higher pressures than what would be normally required. A rule of thumb for systems in the 100 psig range is: for every 2 psi increase in discharge pressure, energy consumption will increase by approximately 1 percent at full output flow. This chapter deals with the subject of pressure drops and what you can do to reduce and minimize them.
Problematic areas for pressure drop include aftercoolers, filters, water separators, dryers, pipes and check valves. Flow restrictions of any type in a system require higher operating pressures, resulting in higher energy consumption.
In general, a properly designed compressed air system should have a pressure loss (or drop) of much less than 10 percent of the compressor's discharge pressure, measured from the point of discharge to the point of end use.
Ways to Minimize Pressure Drops
The following list discusses some common ways to minimize pressure drops in a compressed air system:
- Equipment components for air treatment including aftercoolers, moisture separators, dryers, and filters should be selected with the lowest practical pressure drop at specified maximum operating conditions. Once installed, the manufacturer's recommended maintenance procedures should be followed and documented.
- Maintain air filtering and drying equipment to manage the impact of moisture, e.g. pipe corrosion.
- Properly design the distribution system with appropriate pipe diameter size and looped system configurations where possible.
- Reduce the distance the air travels through the distribution system.
In cases where the air compressor's discharge pressure can be reduced, energy savings can be realized. Before lowering compressor discharge pressure, it is important to check with end use equipment specifications to determine the minimum pressure required by air tools and equipment for proper operations.
- Assess the pressure level requirements of the end-use applications. Minimize the compressed air system pressure to match the end use requirements.
- Check the air pressure at the inlet to the air tools to determine if sufficient pressure is being supplied. It is not uncommon to measure 30 to 40 psi pressure drop between the distribution header drop and the end use. This pressure drop is commonly caused by undersized air lines, quick couplers, filters, regulators and lubricators. Significant pressure loss is also common in end use hoses. Often, there will be a long coiled hose, or a series of hoses, supplying the end use. The resulting high pressure differential will negatively affect the power available to do useful work and often forces main system pressures to rise.
- Examine each end use point and determine the one having the highest pressure requirements. Reduce this pressure to the amount to maintain functionality, and then lower the overall system pressure.
- Specify pressure regulators, lubricators, hoses, and connections with the lowest pressure differential and the best performance characteristics. Size components for the actual flow rates, and not the average flow rates.
End use tool pressure differential can be easily diagnosed by simply making up a test gauge setup that is inserted using quick couplers in the air feed near the end use. Comparing the air pressure with or without the end use consuming air will show the pressure differential.
- Use larger size couplings for reduced pressure differential. For example, at the same flow, a 3/8 inch quick coupler has one-sixth the pressure differential of a 1/4 inch connector.
Minimizing Compressed Air End Use Energy Requirements
Here are some tips to help you to minimize the total energy requirements of your compressed air system.
- Replace inappropriate end use applications (such as open blowing) with efficient models (vortex nozzles, atomizers).
- Install a flow controller to lower plant pressure and reduce artificial demand caused by higher than required pressures.
- Turn off air consuming equipment, using electric solenoids or manual shutoff valves.
- Avoid operation of air tools without a load, as this consumes more air than a tool under load.
- Replace worn tools, as they often require higher pressure and consume excess compressed air than tools in good shape.
- Lubricate air tools as recommended by the manufacturer. Keep air used by all end uses free of condensate in order to maximize tool life and effectiveness.
- Where possible and practical, group end use air equipment that has similar air requirements of pressure and air quality.
Air Compressor Heat Recovery
Approximately 80 percent of the electrical energy used by an industrial air compressor is converted into heat. For many facilities, a properly designed heat recovery unit can recover 50-90 percent of this available thermal energy to offset space and water heating. For new or expanded compressed air systems, the heat recovery potential should influence the final location of the air compressor within the facility.
Heating Air
Packaged rotary screw compressors are ideal candidates for heat recovery for space heating. Generally, ambient air is heated by passing it across the compressor's aftercooler and lubricant cooler. As packaged compressors are enclosed in cabinets, and generally come equipped with heat exchangers and fans, only ducting and HVAC fans need to be installed to extract heat. The ducting can include a vent that is controlled by a thermostat. The vent could direct heated air to the outside during warmer parts of the year. As an energy efficiency measure, approximately 50,000 BTU per hour of heat can be extracted for each 100 scfm air for a compressor operating at full load.
It is not uncommon to be able to heat air to 15 to 25°C above the cooling air inlet temperature with 80-90 percent heat recovery efficiency. It is important to realize in using this heat that any heat recovery ventilation duct must not restrict the compressor cooling air flow. Booster fans are usually required if extensive ductwork is installed.
Heating Water
With an appropriate heat exchanger, waste heat can be extracted from the lubricant coolers in packaged water cooled, reciprocating or rotary screw compressors. Some manufacturers offer this as optional equipment. This can be used to produce hot water for use in central heating or boiler systems, industrial cleaning processes, plating operations, heat pumps, laundries, or any other application where hot water is required. Heat exchangers also offer an opportunity to produce both hot air and hot water, and allow the operator some ability to vary the hot air/hot water ratio. As many water cooled compressors are large (>100 HP), heat recovery for space heating can be an attractive opportunity.
Typically, 50-60 percent of compressor heat can be practically recovered for water heating applications. It is important to realize in using this heat that any heat recovery strategy must not restrict the compressor cooling water flow or overheating could occur.
Implement More Efficient Compressor Control
The discussion in a previous section titled "Compressor Controls and System Performance" showed that significant power can be saved by running compressors in more efficient operation modes. This should be considered for systems that have existing compressors that are capable of such operation. Local compressor service providers can assist in upgrading or modifying controls for more efficient service.
With regard to compressor control the following additional points should be considered:
- For optimal energy and operational performance, systems with multiple compressors require more advanced controls or control strategies (cascaded pressure bands, network or system master controls) to coordinate compressor operation and air delivery to the system.
- Remember to consider the element of time when designing or tuning a compressor control system. Compressors require time to start up and be brought up to speed. This may require extra storage receiver capacity.
- Cascaded pressure bands single pressure bands need to be adjusted from time to time.
- The "trim compressor" should be the one most capable of running efficiently at partial loads.
Install Storage Capacity
Receivers can help compressed air systems operate more efficiently and can help stabilize system pressures as discussed in "Receivers and Air Storage".
The following points should also be considered:
- Where practical, locate the receivers as close to the air compressors as possible.
- For most facilities with load/unload rotary screw compressors, install air receiver capacity of 10 US gallons per cfm of compressor capacity.
- When receivers are exposed to subfreezing temperatures, precautions need to be taken to prevent freezing in the condensate drains. In some cases receivers rated for lower temperatures are required.
- Select a slightly larger receiver than what may be currently required. This will generally result in improvements to stabilizing system pressure and also respond to intermittent demands.
- In cases where the air needs to be dried, it is sometimes beneficial to install two receivers - one before and one after the dryer.
Optimize Air Dryers
Air dryers can consume significant compressed air or electrical power and often have limited turndown capabilities as discussed in "Air Dryers" on page 43. It is possible the existing air dryer could be upgraded or replaced with good savings results. Consider the following points with regard to dryers:
- For new purchases of refrigerated air dryers always consider the energy savings cycling style.
- Avoid drying the air to a dew point level that is lower than what is needed for a specific application.
- Use energy saving dew point controllers for all types of regenerative desiccant dryers.
Reduce System Drainage
Condensate drains are a common point of compressed air loss. Consider airless drains as replacements for timer drains or manual drains that are partially cracked open. The following points should be considered:
- Where possible, procure condensate drains having a gauge glass. This will provide a visual indicator if the trap malfunctions.
- Regularly test automatic drain traps for proper operation.
- Piping should be sloped slightly downwards and away from the compressors.
- Locate drains at the bottom of main headers in order to allow condensate to collect and flow by gravity.
- Avoid using open manual drain valves.
Purchase a More Efficient Compressor
A good energy management strategy may be to purchase a new more efficient compressor as a replacement for an older existing unit. Often the existing unit can be retired to standby duty, providing backup capacity for increased system reliability. Consider the following when purchasing a compressor:
- Purchase the most energy efficient compressors, including ones equipped with premium efficiency motors.
- In situations involving multiple compressors, operate the base load units at maximum capacity rather than partially loaded.
- Consider purchasing and operating at least one Variable Speed Drive compressor to supply variations in flow above the base load.
- Purchase of a two stage compressor might provide better system efficiency if used as a base compressor
Maintaining Your Compressed Air System
It is important to maintain all equipment in the compressed air according to the manufacturers' specifications. This is especially true for the recommended inspection, testing, maintenance, and service schedules specified in operating manuals.
- Leaks on compressors increase operating costs and interfere with control systems.
- Plugged coolers can cause moisture problems, which in turn may lead to open drainage and higher air flows.
- Oil separator differential increases power consumption.
From an energy efficiency perspective, in many cases, it is wise to maintain equipment more frequently than the recommended intervals. This is especially true for managing air leaks, high pressures, moisture and controls.
Figure 27 lists some maintenance tips (aimed at rotary screw compressor systems) for you to consider.
Remember, that the points shown in Figure 27 do not replace the items listed in manufacturers' manuals.
Frequency or Running Hours | Action |
---|---|
Daily |
|
Weekly |
|
Every 3,000 hours |
|
Every 15,000 hours |
|
Before and After Case Examples
This section describes some real life examples of smart energy efficient compressed air system decisions involving:
- Dramatically reducing desiccant dryer purge air
- Using a very small compressor to supply instrument air on weekends
- Specifying on/off control for a rotary screw compressor
"Before" refers to the existing equipment, operations method, or normal procurement decision.
"After" refers to the energy efficient equipment, operations method or preferred procurement decision.
Case 1: Install Purge Controller, Repair Leaks and Lower Pressure
A medium sized furniture manufacturer has two 100 HP screw compressors running in modulating mode feeding a 1,000 cfm desiccant air dryer operating with uncontrolled purge flow. The system runs 6,000 hours a year and is completely turned off on weekends. Problems with end use pressure have been experienced which resulted in a required increase in compressor discharge pressure. Plant personnel attempted to turn off one air compressor to save power but this resulted in low pressure during peak plant flows in the busy daytime production shift. An audit of the facility was done and found the following data:
Before
- Total compressed air production: 450 cfm
- Total compressor energy consumption: 880,000 kWh
- System specific power: 32.9 kW/100 cfm
- Total electrical cost at 10 cents/kWh: $88,000
- Air dryer purge: 150 cfm
- Leaks: 100 cfm
- Drains: 5 cfm
- Average compressor discharge pressure: 120 psi
- Dryer and filter pressure differential: 10 psi
- End use piping differential: 20 psi.
- Required end use pressure: 80 psi
After
The audit report recommended the following which was implemented by the customer:
- Leak reduction: 50 cfm
- Drainage reduction: 5 cfm
- Plant pressure reduction by 15 psi for a 10% flow reduction: 25 cfm
- Install purge control on dryer with purge reduction: 117 cfm
- Total flow reduction: 197 cfm
- This resulted in a final average compressor loading of 253 cfm
- One compressor could now be turned off and the remaining unit would consume 76 kW x 6000 = 456,000 kWh for a savings of 48%
- Savings with existing compressor modulating control: $42,400 per year.
Further measures are recommended:
- Reduce end use piping differential by 13 psi
- Reduce dryer and filter differential by 7 psi
- Compressor discharge pressure reduction: 20 psi
- Install VSD compressor. VSD compressor average power at 253 cfm@ 100 psi: 50 kW.
This resulted in the following savings:
- New system electrical consumption: 300,000 kWh
- New system specific power: 19.8 kW/100 cfm
- New system electrical cost: $30,000 per year
- Savings: 66%
Additional possible savings:
- Heat recovery
- Convert to refrigerated dryer
- End use reduction
Case 2: Use Smaller Compressor During Off-Hours
The company in the previous example installed a dry fire system requiring 5 cfm of compressed air at all times including weekends. No production occurs during these hours. This air demand requires one 100 HP modulating compressor to run for 2,760 hours extra per year.
Plant load on weekend:
- Dryer purge 150 cfm
- Leakage 100 cfm
- Drains 5 cfm
- Fire system 5 cfm
Before
- Power required for 100 HP modulating compressor at 260 cfm = 76 kW
- Total power per year 76 kWx 2,760 hrs = 209,760 kWh
- Operating cost = $20,976 per year (at $0.10 per kWh)
After
- Use a small 5 HP (4.25 kW) 15 cfm rated reciprocating compressor for only the fire system (isolated with check valve).
Operating consumption:
- 5 cfm load/15 cfm rated x 4.25 kW = 1.42 kW x 2760 hrs = 3,910 kWh
- Annual cost = $391
- Annual savings = $20,585 (98%)
Case 3: On/Off vs. Load/No Load Control
A small automobile dealership is considering upgrading their compressed air system. The current system has a 15 HP reciprocating compressor rated at 50 cfm @ 175 psi. The unit is measured at 13 kW average while running. The unit is installed on an 80 gallon receiver.
The average load at the facility was data logged and it was determined that the average flow is 10 cfm with a peak flow of 50 cfm. The air produced by the compressor is filtered with one particulate filter and one coalescing filter arranged in series, and dried in a 75 cfm direct expansion air dryer with hot gas bypass control consuming 0.88 kW. The pressure drop across the air dryer is 4 psi and the filters pressure differential is 4 psi total. The dryer has a timer drain installed that consumes 2 cfm average. The system operates at an average pressure of 145 psi for 3745 hours per year. Blended power rate is 10 cents per kWh.
The dealership is considering the purchase of a 15 HP screw compressor to replace the ailing reciprocating unit. The compressor salesman indicates a new screw compressor can produce 58 cfm at 150 psi @ 13.5 kW so is therefore more efficient. The plan is to install the compressor on the same 80 gallon receiver, use the same filter and air dryer and operate at 145 psi average pressure.
- Existing compressor consumption = 13 kW x10 cfm /50 cfm rated x 3745 hrs = 9,737 kWh per year x $0.10 per kWh = $974 per year
- Dryer consumption = 0.88 kW x 3745 hrs = 3,296 kWh = $330 per year (Note: It is very common to see these dryers left on all the time 8760 hours per year.)
- Total system cost per year = $1,304.
The new screw compressor will be running in load/unload mode with 1.37 US gallons per cfm storage capacity and a 10 psi pressure band. Due to the pressure drop across the filters and air dryer, the compressor will rapid cycle and remain running for the full operating hours. From the load/unload performance curve (see Figure 7) it can be seen the unit will consume an average of 60% of its full load nameplate power.
Proposed Load/unload with standard dryer
- Proposed compressor consumption = 13.5 kW x.6 x 3745 hrs= 30,335 kWh per year = $3,034 per year.
- Dryer consumption = 0.88 kW x 3745 hrs = 3296 kWh x $0.10 per kWh = $330 per year
- Total system costs = $3,364.
The new screw compressor costs about 2.5 times as much in electricity costs to operate.
Alternative High Efficiency System - On/off operation with measures applied
The following additional measures are applied:
- Pressure differential reduced to 0.5 psi across oversized filters and 3 psi across a new cycling style dryer rated at 0.51 kW.
- Average compressor discharge pressure reduced to 115 psi average. A compressor is selected that shuts off immediately as long as the motor starts are less than 6 per hour.
- Drain reduced to zero by use of an airless drain.
- Storage receiver added to reduce compressor starts to less than 6 per hour for all flows.
- Pressure regulating valve added to reduce shop pressure to a constant 100 psi.
The energy efficiency measures reduce the average air flow and power:
- 2 cfm for the drain reduction
- 2 cfm due to the pressure reduction
- Compressor power consumption reduced by 15% due to 30 psi reduction.
- Dryer power consumption is now proportional to flow.
- Proposed compressor consumption (discharge at lower pressure) = 11.5 kW x 6 cfm/58 rated x3745 hrs = 4,455 kWh per year x $0.10 per kWh = $446 per year.
- Dryer consumption = 0.5 kWx 6 cfm/80 rated cfm x 3745 hrs = 140 kWh x $0.10 per kWh = $14 per year
- Total system costs = $460 per year.
- The efficient system consumes about 35% of the original system and 14% of the load/unload system
Next Steps
Compressed air optimization is an ongoing process. It may seem like a daunting task to get going with your compressed air optimization program.
If you are not sure where to begin, start with the "low hanging fruit". This includes fixing air leaks, lowering system pressures, and optimizing control settings. Once you see results (and electricity savings) with these measures, let others know. Management and fellow employees will want to hear about your success.
With initial results, you will be in a better position to convince management to invest capital dollars, to invest in more effective and efficient compressors, to update the distribution system, and to commit resources towards ongoing leak management. Remember that compressed air is a system - if you change a component or setting, it will have an impact elsewhere. The trick is to keep on rebalancing the system to continuously improve the system.
Always factor in the lifetime operating cost when designing or purchasing compressed air system parts. Work with your purchasing department to procure the most energy efficient components, even if these cost more initially. Get others involved at your facility. Let them know that compressed air is not free. Have workers report air leaks. Give maintenance sufficient resources to repair air leaks. Make it a team effort.
Contact your local utility for additional advice and tips for energy efficiency and for help with metering your system.
Glossary
- Absolute Pressure
- Total pressure measured from zero (perfect vacuum).
- Actual cubic feet per minute (acfm)
- Flow rate of air at actual operating pressure and temperature.
- Aftercooler
- A heat exchanger used for cooling air discharged from a compressor. Resulting condensate may be removed by a moisture separator following the aftercooler.
- Atmospheric Pressure
- The measured ambient pressure for a specific location and altitude.
- Automatic Sequencer
- A device that operates compressors in sequence according to a programmed schedule.
- Brake Horsepower
- Horsepower delivered to the output shaft of a motor or engine, or the horsepower required at the compressor shaft to perform work.
- Capacity
- The amount of air flow delivered under specific conditions, usually expressed in cubic feet per minute (cfm).
- Check Valve
- A valve which permits flow in only one direction.
- Compression Ratio
- The ratio of the absolute discharge pressure to the absolute inlet pressure.
- Cubic Feet per Minute (cfm)
- Volumetric air flow rate per minute converted back to ambient conditions.
- Cycle
- The series of steps that a compressor with unloading performs 1) fully loaded; 2) modulating (for compressors with modulating control); 3) unloaded; and 4) idle.
- Cycle Time
- Amount of time for a compressor to complete one cycle.
- Dew Point
- The temperature at which moisture in the air will begin to condense if the air is cooled at constant pressure. At this point the relative humidity is 100 percent.
- Discharge Pressure
- The pressure at the discharge connection of a compressor.
- Discharge Temperature
- The temperature at the discharge flange of the compressor.
- Filters
- Devices for separating and removing particulate matter, moisture or entrained lubricant from air.
- Full Load
- Air compressor operation at full speed with a fully open inlet and discharge delivering maximum air flow.
- Gauge Pressure
- The pressure determined by most instruments and gauges, usually expressed in psig. Barometric pressure must be considered to obtain true or absolute pressure.
- Intercooling
- The removal of heat from air or gas between compressor stages.
- Leak
- An unintended loss of compressed air to ambient conditions.
- Load/Unload Control
- Control method that allows the compressor to run at full-load or at no-load while the driver remains at a constant speed.
- Modulating Control
- System which adapts to varying demand by throttling the compressor inlet proportionally to the demand.
- Pneumatic Tools
- Tools that operate by air pressure.
- Positive Displacement Compressors
- Compressors in which successive volumes of air or gas are confined within a closed space and the space mechanically reduced, resulting in compression. These may be reciprocating or rotating.
- Pressure
- Force per unit area, measured in pounds per square inch (psi) or kilo Pascals (kPa).
- Pressure Drop
- Loss of pressure in a compressed air system or component due to friction or restriction.
- Receiver
- A vessel or tank used for storage of gas under pressure. In a large compressed air system there may be primary and secondary receivers.
- Reciprocating Compressor
- Compressor in which the compressing element is a piston having a reciprocating motion in a cylinder.
- Sequence
- The order in which compressors are brought online.
- Stages
- A series of steps in the compression of air or a gas. Most plant industrial compressors have 2 stages.
- Standard cfm
- Flow of free air measured and converted to a standard set of reference conditions (14.5 psia, 68°F, and 0 percent relative humidity).
- Start/Stop Control
- A system in which air supply is matched to demand by the starting and stopping of the unit.
- Variable Speed Drive
- An electronic device to vary the speed of a motor enabling it to achieve energy savings at low flows.
- Unload/No load
- Compressor operation in which no air is delivered because the intake is closed or modified not to allow inlet air to be trapped.
Literature and Web References
Reference Books
- Natural Resources Canada. “Compressors and Turbines” ISBN 0-662-14166-0, 1987
- Scales, William. “Best Practices for Compressed Air Systems”, 2003
- US Department of Energy. “Improving Compressed Air System Performance” DEO/GO-102003-1822, 2003
Internet References (June 2007)
- BC Hydro Compressed Air Program www.bchydro.com/powersmart/industrial.html
- Compressed Air Challenge www.compressedairchallenge.org
- Compressed Air & Gas Institute www.cagi.org
- Hydro Quebec Compressed Air www.hydroquebec.com/business/appui_pmi/procedures_outils/compression.html
- Manitoba Hydro Compressed Air Program www.hydro.mb.ca/pop/compressed_air.shtml
- Natural Resources Canada, Office of Energy Efficiency http://www.nrcan.gc.ca/sites/nrcan/files/oee/pdf/publications/industrial/cipec/compressed-air.pdf
Useful Measurement and Conversion Factors
Temperature
Fahrenheit Temperature = (1.8 × Celsius Temperature) + 32
Pressure
1 bar = 14.50 lbf/sq.in (psi)
Length
1 cm = 0.3937 inches
1 m = 3.28 feet
Volume
1 liter = 0.21997 gallons (Imperial)
1 liter = 0.26417 gallons (US)
Energy
1 kWh = 3.6 MJ
1 kWh = 3,412 Btu
Power
1 HP = 0.746 kW
Quick Tips to Optimize Compressed Air Systems
Here's a list of ten things you can do, continue to do, or evaluate in order to kick start your compressed air optimization and energy efficiency program:
- Identify and repair air leaks, starting with the biggest.
- Measure and baseline your compressed air system to determine the operating costs and efficiency.
- Operate compressed air system at the lowest practical pressure.
- Install, adjust and maintain automatic system controls to coordinate operations of air compressors.
- Turn off compressed air supply to zones, equipment and applications that are not operating.
- Use a blower rather than a compressor where appropriate.
- Use air receivers to reduce compressor cycling and respond to peak air demands.
- Use larger diameter pipes and looped air distribution configurations where practical.
- Maintain compressed air equipment, filters and drains at an appropriate level.
- Constantly ask if compressed air is the best utility for the intended application.
Remember to involve compressed air operating and maintenance personnel in production and end use decisions.
Your feedback and comments are appreciated.
Please provide suggestions to:
Page details
- Date modified: