Intelligent Net-zero Energy Buildings
Lead Proponent: Concordia University
Location: Montreal, QC
ecoEII Contribution: $ 1,000,000
Project Total: $ 1,731,000
Project Background:
Smart Net-zero Energy Buildings strategic Research Network (SNEBRN) brings together 29 Canadian researchers from 15 universities to develop smart net-zero energy homes and buildings of the future. The Network was awarded $5M over 5 years (2011-2016) from Natural Sciences and Engineering Research Council (NSERC) to perform research that would facilitate widespread adoption of net-zero energy buildings (NZEB) design and operation concepts suited to Canadian climatic conditions and construction practices by 2030, in key regions of Canada. Whereas SNEBRN’s program activities focus on the development of general modelling and methodologies, the project “Intelligent Net-zero Energy Buildings” involves complementary activities not funded by NSERC funds. Concordia University, the project proponent, and its network of university partners were awarded $1M from ecoEII to conduct research on selected promising technologies that would enable net-zero energy homes and buildings.
Results:
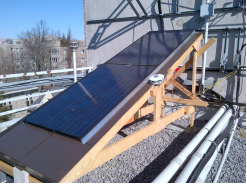
PVT collectors on simulated roof
Larger image
Text version
An experimental test apparatus was constructed using two 235W solar panels. The panels were mounted at a slope of 22.5 degrees in a column directly on a south facing, simulated roof section. Horizontal slots were cut through the upper and lower frame sections of the PV modules to allow air to circulate behind the PV panel by natural or forced convection. During testing, ambient air was drawn through the flow passage (i.e., air-channel) formed by the PV panel and the roof section.
In order to achieve NZEB, the envelope needs to be energy positive and generate solar electricity and heat. Novel building-integrated photovoltaic/thermal (BIPV/T) prototypes were designed, built and tested in Concordia’s Solar Simulator Environmental Chamber (SSEC) lab. The new façade BIPV/T designs used distributed inlets instead of unglazed transpired collectors (UTC). Test results indicated an overall 10% improvement in thermal performance when two inlets (instead of one) were used, specifically with a semi-transparent (instead of black opaque) back sheet on the PV.
A model for fenestration systems with two controllable layers for solaria/greenhouses was developed. A test-room prototype was then built to validate the model and used to develop and verify control strategies that enhance solar energy utilization. It included controlled motorized interior and exterior shades, controlled heating and phase change material (PCM) in the back wall. The PCM was used as both passive and active thermal storage. The completed prototype with integrated PCM wall was tested in the SSEC lab.
In a photovoltaic thermal (PVT) hybrid system, PV modules are ventilated by natural or forced airflow to reduce panel temperatures, and the heated air is then used for space and water heating, or to preheat ventilation air. An experimental PVT apparatus was constructed and its performance tested. Results indicated that any level of airflow behind the panel, including natural convection, increased the electrical output when compared with closed vents (i.e., stagnation condition). However, using a fan to increase forced air flow above 30 cfm had a negative effect on net electrical output, as less electricity is produced than is consumed by the fan. On the other hand, thermal collectors in PVT systems need high temperatures (e.g. > 60°C) in order to provide useful thermal energy. A heat pump can be used to deliver heat captured from the PV array to a thermal load or a thermal storage. Queen’s University developed an experimental test rig to evaluate the performance of liquid-based heat pumps. Testing indicated that the R410-A heat pump operates well at low source temperatures but struggles to deliver heat at 60°C. The trans-critical CO2 heat pump could deliver high temperatures (>90°C) but at the cost of increased complexity.
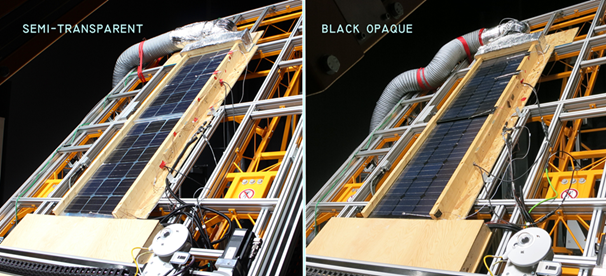
BIPV/T façade under test at SSEC, both with two inlets; left with semi-transparent back sheet, right with black opaque back sheet
Larger image
Text version
Custom BIPV/T prototypes were designed and constructed at Concordia's SSEC lab using different designs of frameless photovoltaic panels specially built at the Canadian Solar factory with the participation of Concordia University researchers and students. A manifold is connected at the top of the two prototypes shown to test thermal and electrical efficiencies under different air flow rates and solar radiation intensities produced by the solar simulator.
The design of geothermal bore fields relies on the determination of long-term thermal response factors, known as g-functions, which are obtained from numerical or analytical models. Using dimensional analysis to reduce testing time, a small-scale borehole prototype was built to validate these models experimentally. Testing of the small scale prototype in an existing sand reservoir produced results that constituted the first known experimental validation of g-functions. A second borehole prototype (four-pipe, dual U-tube) was built and used to validate new numerical models.
Heuristic predictive control algorithms and strategies that reduce energy consumption and peak demand were developed and tested on a building in Concordia University, where a hybrid ventilation system and automated motorized shading were installed.
Benefits to Canada:
Once adopted, the novel NZEB concepts, models, design techniques, and control strategies proposed in this Project will help to reduce energy consumption and improve energy efficiency of homes and buildings across Canada.
Next Steps:
Continue to advance research in NZEBs and enabling technologies, and train highly qualified professionals in order to transform both the buildings and energy industries.
Page details
- Date modified: