Implications for Economic Development and Adaptation within Key Sectors
Back ¦ Chapter Index ¦ Next Page
4.1 HYDROELECTRIC DEVELOPMENT
Demand for electricity is rising in all three territories, due to increasing population and heavy industry, such as diamond mines in the Northwest Territories and Nunavut. Alternative renewable energy sources, such as solar, wind, wood and even geothermal power, could help to meet some of the increasing demands for electricity, and territorial government agencies have indicated that they are committed to increasing renewable energy supply. There is already a significant dependence on hydroelectric generation, with seven large (>10 m in height) hydroelectric dams operating in the Yukon and Northwest Territories (Canadian Dam Association, 2003), along with a range of small, often privately owned, micro-hydro facilities. Further expansion of micro-hydro facilities is under consideration, but the major northern rivers still offer some of the largest potential (Prowse et al., 2004).
Changing climate will affect the capacity and operations of current and future hydroelectric developments, as well as affecting the demand for electricity. Projected increases in winter runoff from rainfall and enhanced winter snowmelt will lead to a decline in winter snow storage. Reservoir capacities on current and future developments may need to be expanded to offset this loss in natural storage. For some sites, this could be achieved by raising the height of the retention dam, thereby increasing storage area and volume. Where the landscape (e.g. relatively low relief) or operations (e.g. run-of-river power plants) preclude such an approach, adaptation measures may involve the construction of additional storage or facilities in other locations. The gradual loss of meltwater contributions from glaciers as they ablate and retreat will also need to be factored into future calculations of capacity.
Changes in the magnitude and seasonality of flows will also necessitate an increased focus on the safety of existing dam structures (World Commission on Dams, 2000). Of particular importance is a need to redefine the Inflow Design Flood (IDF: volume, peak, duration, shape and timing), commonly defined as the most severe inflow flood for which a dam and its associated facilities are designed (Zielinski, 2001). Concern that changing hydrological conditions will require reassessment of IDFs has already been noted for hydroelectric operations on the Snare River (Bruce et al., 2000). Changes in river-ice regimes will alter threats from downstream ice jamming (Prowse and Beltaos, 2002). Fuller assessment of the risks to hydroelectric facilities under a changing climate, however, requires hydrological models capable of predicting flow in northern regions that often lack flow gauges (e.g. Spence et al., 2005).
4.2 OIL AND GAS
The oil and gas industry involves exploration, extraction, production and delivery. Although changes in climate need to be considered for all four, exploration activities are likely to be most affected. In 2006, there were active and potential exploration activities in the Eagle Plains area of the Yukon and in the Cameron Hills, Fort Liard and Mackenzie Delta areas of the Northwest Territories. Some of the largest future potential reserves exist within the Canadian Arctic Archipelago (e.g. Drummond, 2006), and projected decreases in sea-ice cover may result in this area becoming a focus of additional exploration activity.
Thawing of permafrost and changes in snow cover will necessitate an increased focus on low-impact vehicles and/or changes in seasonal scheduling of exploration activities. The unpredictability of the winter season and the winter ice-road system will necessitate greater flexibility in scheduling of exploration and extraction activities. The greatest impact of changing climate on exploration, however, may relate to the use of in-ground sumps for drilling wastes. Disposal in sumps relies on the presence of permafrost to prevent subsurface movement of drilling wastes into the surrounding environment (French, 1980; Dyke, 2001). Increased ground temperatures resulting from increases in air temperature and/or snow depth (Jenkins et al., 2005) will increase the likelihood of contaminant transport. Alternate drilling-waste practices, including remote sumps, central processing facilities, down-hole injection or transportation of waste to outside the territories (e.g. Environmental Studies Research Funds, 2004), represent potential adaptations to climate change.
Offshore exploration drilling, such as that recently conducted by Devon Canada Corporation in the Beaufort Sea, will be affected by decreasing sea-ice cover. Future development may require design changes to drilling platforms to counter the effects of increased wave action and storm surges. One possible adaptation would be to increase the use of exploration drill ships (Croasdale, 1993).
Processing plants, one of the largest infrastructure components of production, must maintain their structural integrity over the multi-decade lifetime of a project. Design of production facilities needs to consider the effects of climate change on permafrost and ground stability. For facilities located on river channels or coasts, such as in the Mackenzie Delta region, additional factors such as river-ice break-up and ice-jam flooding, coastal erosion and sea-level rise must be considered. One recently proposed method to avoid some potential impacts involved using a barge for production facilities, rather than a land-based facility.
Oil and gas are delivered to markets through pipelines that are designed according to environmental conditions, many of which are influenced by climate. Currently there are three small pipelines operating in the North, but Imperial Oil Resources Ventures Limited Canada (Imperial Oil Resources Ventures Limited, 2004) submitted an application in 2004, as part of the Mackenzie Gas Project, to construct a large diameter (30-inch), high-pressure chilled pipeline to deliver natural gas from three fields in the Mackenzie Delta to northern Alberta. If approved, the Mackenzie Gas Project would be the largest industrial development in the Canadian Arctic. A number of geotechnical –climate change issues need to be addressed when constructing pipelines in permafrost zones, such as changes in the ground thermal regime, drainage and terrain stability, all of which may result from a warming climate over the lifetime of such a project (see Section 4.4). Experience from the Norman Wells pipeline illustrates the need to closely monitor the performance of both pipeline and right-of-way to determine if actions are required to maintain pipeline integrity and to minimize environmental impacts (e.g. Agra Earth and Environmental Limited and Nixon Geotech Ltd., 1999; Nixon and Burgess, 1999; Oswell, 2002). Adaptation, such as adding insulation or using thermosyphons to induce artificial cooling, may be required, especially along sensitive slopes.
4.3 MINING
There are currently three major mines operating in the northern territories: two diamond mines in the Northwest Territories and one diamond mine in Nunavut. Other projects have recently been approved or are in the advanced stages of environmental assessment and regulatory process in all three territories. Moreover, development of integrated land and marine transportation networks in response to projected declines in sea-ice cover is likely to stimulate further mine exploration and development (see Case Study 1). The principal mineral deposits include diamonds, gold, tungsten, silver, lead, iron, copper, zinc, nickel, coal, tantalum, niobium, lithium, cobalt, bismuth, uranium, beryllium and barium.
Resupply of existing mines is generally limited to winter periods and the availability of ice roads, whereas exploration activities are usually restricted to short summer periods, with access by air. Climate-change should be considered in the engineering design, during operations and in final closure and abandonment of mines, a planning process termed “designing for closure” (Milburn and Brodie, 2003). Of particular concern for mine access is the expected reduction in the availability of ice roads, which may necessitate development of all-season roads and/or water-based transportation systems (see Section 4.5.2). Another concern is the impact of climate change on permafrost and ground stability (see Section 4.4). The stability of waste-rock piles, tailings piles and tailings-containment impoundments often depends on maintenance of frozen conditions to ensure that contaminants and acid-metal leachate (or acid-rock drainage) are not discharged to the environment (Mine Environmental Neutral Drainage Program, 1997).
4.4 INFRASTRUCTURE
Permafrost and the ground ice it contains present challenges for the design, construction and operation of infrastructure in northern Canada (e.g. Smith et al., 2001a; Couture et al., 2003) and throughout the circumpolar region (Instanes et al., 2005). Although ice-bonded frozen ground can provide a strong foundation for infrastructure, thawing of the ground leads to loss of strength, as well as to settlement and instability. The removal of insulating vegetation/organic cover and other disturbances of the ground surface that generally accompany construction can significantly alter the ground thermal regime, resulting in warming and thawing of permafrost. Additional warming may occur due to heat generated by the structure itself (e.g. heated buildings and buried water, sewage or hydrocarbon pipelines). For larger structures, particularly such linear structures as runways, roads and pipelines that cover large distances, concerns include differential settlement due to spatial variations in soil characteristics and ice content, and slope instability resulting from permafrost thawing.
Climate warming presents an additional challenge for northern development and infrastructure design. In the short term (years), the impacts associated with ground disturbance and construction will be of far greater significance than those related to climate. The impacts of changing climate become increasingly significant over longer time scales (decades). For example, permafrost monitoring along the Norman Wells pipeline corridor has shown that the climate signal is largely obscured by the effect of disturbance of the ground surface related to right-of-way clearing (Burgess and Smith, 2003). This was especially true in the first 5 to 10 years following the disturbance, when permafrost was responding to an abrupt change in ground-surface temperature of 2 to 4 °C, and large increases in thaw depth occurred. In contrast, changes in ground thermal regimes related to climate occur much more slowly, with changes in ground-surface temperature on the order of 2 to 4 °C occurring gradually over a period of several decades.
Failure to take proper precautions in the engineering design of infrastructure in permafrost regions can have serious consequences. This was the case in the Yukon in the 1890s, when construction in Dawson proceeded with no consideration for the ice-rich alluvial sediments that underlay the site (Hughes, 1989). Subsequent thawing of ground ice and associated settlement damaged buildings, causing many to become uninhabitable, and made many roads impassable. Until the middle of the twentieth century, the presence of permafrost was often not recognized. Current engineering practices, however, have the objective of minimizing both disturbance to the terrain and the impacts on structures. Locations are generally chosen to avoid thaw-sensitive soils, and modern infrastructure is designed to preserve thaw-sensitive permafrost, limit thaw settlement and withstand thaw settlement where it does occur.
CASE STUDY 1
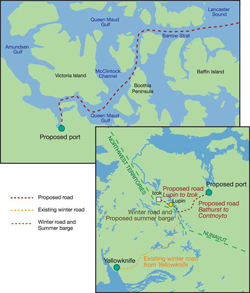
FIGURE 14: Proposed marine and land transportation network in northern Canada, consisting of a) shipping route to new port at Bathurst Inlet, showing average date of ice break-up; b) road network linking the port to resource developments in Nunavut (Bathurst Inlet Port and Road Joint Venture Ltd., 2003, 2007).
Opportunities for Growth in the Mining and Transportation Sectors
Reductions in the duration and extent of sea-ice cover as a result of changing climate present new opportunities for marine transport in the Canadian Arctic (see Case Study 2). These opportunities, in turn, will potentially increase the competitiveness of both existing and planned resource development. Recent expansion of large-scale gold, base metal and diamond projects in the Slave geological province of the Northwest Territories and Nunavut, in concert with the prospect of a longer shipping season, greatly enhance the economic rationale for construction of a deep-water port along Canada's northern coastline. Such a port would ideally be fed by an all-weather road network, linking it with the inland mine sites.
One proposal currently under consideration calls for development of a port at the southern end of Bathurst Inlet, NU, capable of handling 50 000-ton vessels and smaller barges serving western Nunavut (Kitikmeot) communities (Figure 14; Kunuk and Stephens, 2003). The port development would be augmented by 211 km of all-weather gravel roads connecting Bathurst to Contwoyto (Figure 14). The economic benefits of such mining activities could be substantial (Kunuk and Stephens, 2003). Moreover, development of the port and road infrastructure would likely stimulate additional development at known mineral deposits along the transportation corridor, attract new mineral exploration and link Nunavut communities to an enhanced Arctic Ocean marine traffic system.
In the past, climate warming was not considered in engineering design, even for such large projects as the Norman Wells pipeline, which was designed in the early 1980s. Climate warming, however, has increasingly been recognized as a critical factor over the lifetime of major infrastructure projects in northern Canada, and has been incorporated in the engineering design and environmental impact assessments of such developments since the late 1990s. A risk-based project screening tool has been developed for considering climate change in engineered facilities and for gauging the level of analysis required for a particular facility (Environment Canada, 1998). A key factor in the screening process is the consequence of failure, so that the level of analysis is higher where the potential consequences of failure are greater (e.g. for a waste-containment facility).
For significant recent projects, such as water-retention or tailings-containment structures, large buildings and linear infrastructure such as pipelines and roads, climate change has been considered in the design phase. Indeed, such consideration is a requirement of the Canadian environmental assessment process (e.g. Lee, 2000; Federal-Provincial-Territorial Committee on Climate Change and Environmental Assessment, 2003). For example, climate warming was recognized as a concern over the lifetime of the Ekati diamond mine, which opened in 1998, and the potential impacts were considered in the design of the mine's frozen-core tailings dams (EBA Engineering Consultants Ltd., 1995). Other recent mining projects in the Northwest Territories and Nunavut, as well as the proposed Mackenzie Gas Pipeline, have considered climate warming in both their engineering design and environmental-impact assessment. Design of recently constructed large buildings, such as the Inuvik Regional Health Centre, has considered the impact of climate warming on the underlying permafrost (Hayley, 2004).
For structures built prior to the late 1990s, climate change may cause increased thaw depth and settlement beyond the original design values, potentially resulting in increased maintenance costs and remedial work to ensure structural integrity. Concerns about thaw settlement will be less where soils have low ice content, structures are founded on bedrock or constructed on deep foundations, and for smaller structures built on adjustable foundations. Structures on thaw-sensitive soils built on shallow foundations are at greatest risk. A pilot study of Norman Wells and Tuktoyaktuk, NT compiled digital databases of all available borehole geotechnical data and an inventory of infrastructure and foundation systems, to identify those structures where impacts of climate warming may be of concern (Couture et al., 2000, 2001; Chartrand et al., 2002). Similar databases could be compiled for other communities to aid climate change adaptation planning for existing structures, and to facilitate future land-use planning.
Of greatest concern in the context of changing climate are structures that need to maintain their integrity over periods of many decades to centuries, and/or have significant consequences associated with failure. Waste-containment facilities present a particular challenge, as facilities constructed several decades ago were not designed for the warmer conditions presently being experienced, and certainly did not consider the warmer conditions projected for the future. Failure of frozen-core dams on tailings ponds due to thawing and differential settlement, or thawing of tailings piles associated with climate warming, could result in contaminants being released into the surrounding environment, with subsequent impacts on ecosystems and human health. Remedial action may be required at these older sites, possibly including the use of thermosyphons to ensure the maintenance of frozen conditions, or modification to covers of land-based tailings piles to ensure that the tailings remain encapsulated in permafrost (e.g. BGC Engineering Ltd., 2003; Mine Environmental Neutral Drainage Program, 2004). New containment structures in the southern part of the continuous permafrost zone may need to consider techniques presently used in the discontinuous permafrost zone or in non-permafrost areas, such as the use of impermeable liners.
Adaptation of northern infrastructure to climate change will largely involve approaches already in use to reduce the impacts of ground disturbance. These include the use of pile foundations (that may need to be deeper to account for climate change), insulation of the surface (which may require thicker gravel pads), clearance of snow (to promote colder winter ground temperatures), adjustable foundations for smaller structures, and increased use of artificial cooling to ensure that foundation soils remain frozen (Couture et al., 2003). Recently developed techniques, such as air-convection embankments (Goering, 1998, 2003), may also be utilized. Where permafrost is thin, frozen ice-rich material may be excavated and replaced with thaw-stable material, or intentionally thawed by clearing vegetation and postponing construction for several years until the permafrost has completely degraded and the ground has settled (cf. Brown, 1970).
Finally, an important element of any adaptive response will be monitoring to: 1) evaluate infrastructure performance; 2) determine if changes in permafrost conditions deviate from those predicted; and 3) decide whether additional adaptation measures are required.
4.5 TRANSPORTATION
4.5.1 Marine Traffic
The many different types of sea ice and glacier ice in Arctic marine environments, in addition to such conventional factors as storm waves and shoals, present unique risks to transport. In turn, marine transport presents potential risks to the Arctic environment, including the possibility of fuel and cargo spills, disturbance of wildlife via vessel presence and underwater and airborne noise, and destabilization of fast ice that can disrupt both animal and human travel. The Canadian Arctic provides three routes for marine shipping: to the port of Churchill and other communities in Hudson Bay via Hudson Strait; to the Beaufort Sea via Bering Strait or the Mackenzie River; and through the Arctic Archipelago via the Northwest Passage. The Northwest Passage extends from Baffin Bay through Lancaster Sound to the Beaufort Sea via a number of alternative waterways (Figure 15; Case Study 2), including:
- the Viscount Melville Sound–M'Clure Strait route, which is the most heavily choked with ice;
- the Viscount Melville Sound–Prince of Wales Strait route, which is the next most difficult, but would be the preferred route for deep-draft vessels; this is the route that was taken by the SS Manhattan and the USCGC Polar Star; and
- the Prince Regent Inlet–Larsen Sound–Victoria Strait–Coronation Gulf route, which is the one most commonly used by Canadian Coast Guard icebreakers, commercial ships and smaller vessels, despite the somewhat narrow and difficult passage through Bellot Strait and the generally shallower waters; the route via Pell Sound to Larsen Sound provides an alternative should Prince Regent Inlet be ice bound; the most challenging ice conditions along either of these routes are usually found in Victoria Strait.
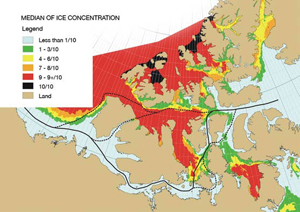
FIGURE 15: Typical routes for the Northwest Passage, superimposed on charted median ice concentration (1971-2000) for September 3. Colour indicates ice concentration in tenths (courtesy of Humfrey Melling, Fisheries and Oceans Canada).
Another route through the Arctic Archipelago, not normally considered to be part of the NWP, is through Hudson Strait, Foxe Basin, Gulf of Boothia and Bellot Strait to Larsen Sound. Although strong tidal currents present navigational challenges in Fury and Hecla Strait, reduced ice in Foxe Basin and the much shorter sailing distance from the central Arctic to the south have made this an attractive route in recent years (J. Falkingham, Environment Canada, pers. comm, March 20, 2007).
Arctic seaways are used for the resupply of communities, export of raw materials and cruises for sovereignty, tourism or science. Resupply of communities generates the most predictable traffic. Ocean-going ships are generally used for the eastern sea-lift, while tugs and barges are better suited to the shallow waters of the western Canadian Arctic. Raw materials have been exported at times during the last 25 years. Although this type of use is presently dormant, there are expectations that at least two high-grade iron mines could start production and export of concentrate in the next few years. Scientific cruises to the Arctic have increased dramatically since 1990, and are frequently multitasked on Canadian Coast Guard (CCG) icebreakers that simultaneously serve other roles, such as navigational support and maintaining a Canadian government presence. In 2004, the CCGS Amundsen was outfitted specifically to support research on the impacts of climate change in the coastal Canadian Arctic, as part of the ArcticNet Network Centre of Excellence (ArcticNet, 2007), and now regularly traverses the Northwest Passage. Similarly, tourist cruises through the passage are becoming increasingly common. Although international shipping represents another usage of marine waterways, initiatives by the tanker Manhattan in 1969 and 1970 revealed the serious challenges to safe, cost-effective and predictable transshipment through the Northwest Passage.
Arctic Canada has never seen year-round shipping, nor is it expected to for decades to come (Wilson et al., 2004). Resupply presently begins in July and ends in October, and frequently requires support from Canadian Coast Guard icebreakers even within that window. Winter shipping is difficult relative to summer shipping due to that fact that winter ice is colder, and therefore stronger, than summer ice. In addition, winter ice is consolidated from shore to shore, without the cracks (leads) that make it easier for a ship to pass through. Additionally, near-total darkness and bitter cold make winter navigation exceedingly hazardous. Multi-year ice, which does not soften much in summer, is a serious hazard to ships year round. At high concentrations, multi-year ice is a barrier to all but the most powerful icebreakers, even in summer. In winter, it is effectively impenetrable.
The most obvious impact of changing climate on Arctic marine transportation will be an increase in the length of the summer shipping season, with sea-ice duration expected to be 10 days shorter by 2020 and 20 –30 days shorter by 2080 (Table 8; Loeng et al., 2005), although there is no expectation of an ice-free Arctic in winter. Even though a longer shipping season appears beneficial, ice conditions in all areas of the Canadian Arctic are highly variable from year to year and will likely remain that way. Hence, there will continue to be summers with ice conditions either more benign or far worse in the future.
2020 | 2050 | 2080 | |
---|---|---|---|
Sea ice: | |||
Duration | Shorter by 10 days | Shorter by 15–20 days | Shorter by 20–30 days |
Winter extent | 6–10% reduction | 15–20% reduction | Probable open areas in high Arctic (Barents Sea and possibly Nansen Basin) |
Summer extent | Shelves likely to be ice free | 30–50% reduction from present | 50–100% reduction from present |
Export to North Atlantic | No change | Reduction beginning | Strongly reduced |
Type | Some reduction in multi-year ice, especially on shelves | Significant loss of multi-year ice, with no multi-year ice on shelves | Little or no multi-year ice |
Landfast ice: | |||
Type | Possible thinning and a retreat in southern regions | Probable thinning and further retreat in southern regions | Possible thinning and reduction in extent in all Arctic marine areas |
Future trends in the sea-ice environment of northern Canada will likely differ somewhat from those outlined in Section 3.1 for the Arctic as a whole. The persistent and preferential occurrence of multi-year ice on the Canadian side of the Arctic (Rigor and Wallace, 2004) is likely to present challenges to northern shipping for at least several decades. At present, much of the ice cover of the Canadian Arctic Archipelago is land-locked for up to 10 months each year, and drifts slowly southward from the Arctic Ocean to the Northwest Passage (Melling, 2002). The longer thaw season of a warmer climate will promote a longer period of weakness in the pack, resulting in more rapid drift of Arctic Ocean multi-year ice through the Arctic Archipelago and into the Northwest Passage. This will tend to maintain, or even increase, the hazard to shipping in the Northwest Passage as long as there is a supply of ice from the Arctic Ocean.
Hudson Bay and the Beaufort Sea, because of their very different ice regimes, are both likely to see increased numbers of transits by large ships. A longer summer shipping season will likely encourage shipping through the port of Churchill on Hudson Bay; in the Beaufort Sea, it will increase the appeal of offshore hydrocarbon development and of shipping oil and gas in large ships westward and through Bering Strait. Increased wind fetch will increase risks from waves and surges to barge traffic, coastal infrastructure and small-boat use by northern residents. The increase in marine traffic and some hazards are likely to increase demands for:
- updated navigational charts;
- marine-weather forecasting, including storm waves and surges;
- ice reconnaissance and forecasting;
- icebreaking support services and search-and-rescue capability;
- marine-traffic surveillance, control and enforcement;
- coastal facilities for fuelling and loading cargo;
- ice-class vessels for new sea conditions and cargos (e.g. barges, tugs, tankers and bulk carriers); and
- specialized crews for the Arctic trade.
CASE STUDY 2
The Future of the Northwest Passage
The Northwest Passage is a series of seven major channels running through the islands of Canada's Arctic Archipelago (Figure 15). Considerable debate has emerged during the past two decades regarding the future of the Northwest Passage, mainly because of the projection of future reductions in sea-ice cover. Historically, multi-year sea ice has entered the archipelago via the small channels along the northwestern side of the Queen Elizabeth Islands and has grown in situ in Viscount Melville Sound, to be exported eastwards and westwards (Figure 15; Melling, 2002). In heavy ice years, multi-year pack ice extends southward to the Tuktoyaktuk Peninsula.
An ice-free Northwest Passage would create a significant economic opportunity for shipping companies, as it provides a route that is about 7000 km shorter for travel between Tokyo and London than using the Panama Canal. It also offers the advantage of allowing passage of ships that cannot fit through the canal systems and, for example, are forced to travel around Cape Horn. The capacity to accommodate even supertankers was demonstrated with the 1969 passage of the SS Manhattan (modified for ice breaking service) between the oilfields of Prudhoe Bay and the east coast of the United States. In some instances, the shelter from storms provided by the archipelago, compared to the open Pacific shipping routes, is another advantage of the Northwest Passage, as evidenced by the successful 1999 towing of a dry dock from Vladivostok, Russia eastward through the passage en route to its final destination of Bermuda (Huebert, 2001).
An alternative for cross-Arctic transit, the northern coast of Eurasia, is expected to open before the Northwest Passage, since the remnant ice pack will tend to shift towards North America. Furthermore, significant variability in ice conditions and resulting shipping hazards will likely persist in the Northwest Passage, even in the warmest summers. The persistence of such hazards might reduce interest in use of the Northwest Passage for commercial shipping (e.g. Griffiths, 2003).
While international disputes about Canada's claims to the lands and islands in the Arctic Archipelago were largely resolved in the 1930s, the Northwest Passage remains the focus of international discussion. The two opposing views are that: 1) the Northwest Passage is part of Canada's 'historic internal waters' found within straight baselines enclosing the archipelago, giving the country unfettered authority to establish controls and conditions to protect its safety, security, environmental and Inuit interests; and 2) the Passage is an international strait through Canadian territorial waters where the right to regulate is subject to some limitations. As summarized by Pharand (2007), Canada has exercised jurisdiction over shipping in the Passage through the creation of an arctic shipping regime consisting of a number of laws and regulations (18 in the case of passenger vessels), including the oldest of these laws, the Arctic Waters Pollution Prevention Act (AWPPA) of 1970. Canada led the effort for the inclusion of Article 234, known as the 'Arctic Exception', in the 1982 United Nations Convention of the Law of the Sea (UNCLOS). Article 234 allows coastal states to enforce laws relating to maritime pollution out to 200 nautical miles when virtually year-round ice creates exceptional navigational hazards (Charron, 2005; Barber et al., 2006), and legitimized Canada's authority to enforce a very strict anti-pollution regime. As customary international law developed, Canada also amended its legislation to extend the definition of the territorial sea (and hence coastal state rights) from 3 to 12 nautical miles (Killaby, 2006). This is significant, given that the narrowest point of the Northwest Passage is less than 24 nautical miles across (see also Barber et al., 2006).

As long as ice conditions remain hazardous and unattractive to commercial shipping, there is little incentive for any country to challenge the Canadian position. However, changing climate and associated changes in sea-ice regimes may increase pressure to designate the Northwest Passage an international strait. Enhanced marine traffic through the Northwest Passage is likely to lead to a number of additional issues that will need to be addressed by Canada and northerners. These include the use of northern coastlines for illegal activities (e.g. smuggling), spread of new and exotic species and diseases, and increased marine-traffic accidents and related threats from pollution (e.g. Kelmelis et al., 2005). Adaptive responses to such issues may include increased Arctic surveillance and policing, and additional enforcement of environmental standards and regulations (e.g. Huebert, 2003; Charron, 2005; Barber et al., 2006). The reduction in sea ice and increased marine traffic would have significant negative impacts on the traditional ways of life of northern residents, but also offer opportunities for economic diversification in new service sectors supporting marine shipping. It has even been envisaged that some settlements, such as Tuktoyaktuk and Iqaluit, could become important ports of call in the future (Huebert, 2001), leading to significant socioeconomic changes in these communities.
Climate change is also expected to change the nature of the risks to shipping traffic in many areas of the Arctic. Rather than being confronted with extensive ice pack that necessitates icebreaker escort, ships in the future will see easier navigating conditions in general, punctuated by frequent occurrences of ice pressure in congested straits, multi-year ice in low concentrations that is difficult to detect, and extreme variability of conditions from one year to the next. As such, there will be a need for continued, if not increased, icebreaking support for increased and more broadly dispersed shipping activities.
4.5.2 Freshwater Transport
Historically, open-water transport on rivers and lakes was the main method of transporting goods to northern communities using, for example, the main stem and major tributaries of the Yukon and Mackenzie river systems. The Mackenzie remains a major freshwater transportation system, with goods (largely bulk fuel, equipment and general cargo) that originate from the northern railhead at Hay River being transported via barge –tug boat trains across Great Slave Lake to riverside communities and ultimately to Tuktoyaktuk. Here, barges are uncoupled and transported by ocean-going tugboats to as far east as Taloyoak on the Boothia Peninsula and as far west as Barrow, Alaska.
An increase in the river ice-free season as a result of climate warming will expand the potential period of operation for Mackenzie barges from its current four-month season between mid-June and mid-October. Lonergan et al. (1993), for example, projected that a 6 to 9 week reduction in the ice season could result in a 50% increase in the use of barge-based transport along the Mackenzie, although this figure pales compared to the 600% increase in transport forecast to occur in the next few years as a result of the Mackenzie Gas Project in the Mackenzie Delta (Neudorf, 2005; see Section 4.2). However, these forecasts do not include consideration of how climate-related changes in lake and river levels (cf. Kerr, 1997; Blanken et al., 2000; Rouse et al., 2003) could affect the use of flat-bottomed barges in an already relatively shallow river system, particularly during late summer low-lake and low-flow periods. Upstream flow regulation is another factor influencing low-flow levels and has contributed to increasingly difficult river navigation during the last half century (e.g. Gibson et al., 2006).
Dredging selected portions of the channel may offer localized, short-term solutions to decreased water levels but is unlikely to be a practical long-term adaptive measure, given the rapidly changing bed morphology of the Mackenzie and other northern river systems (e.g. Prowse, 2001). Upstream flow regulation could be used to increase late-summer flows but would, in turn, negatively impact hydroelectric-generating potential. The most obvious adaptation measure to increasingly difficult river transport is an increase in all-season road networks, although there would be significant engineering challenges in constructing and maintaining such roads in permafrost terrain (see Section 4.4).
4.5.3 Winter Roads
Although lake and river ice have historically served as natural transportation routes, and modern engineering has led to increasingly sophisticated methods of winter-road construction and expansion, little scientific literature exists about the effects of climate on these systems. The most relevant information, even about the basic winter-road network, is contained only within consultant and local government reports or news media. Since the 1950s, the seasonal road network has evolved into a large suite of private and public lake and river crossings linking northern communities and all-season road systems. Ice roads and ice bridges that are constructed and maintained each winter provide a relatively inexpensive way to supply northern communities and industry, particularly the rapidly expanding mining sector that relies on ice roads to move heavy equipment, materials and fuel for the remainder of the year. Additionally, they form critical travel routes connecting communities and facilitating the ability to continue social and cultural activities during winter months.
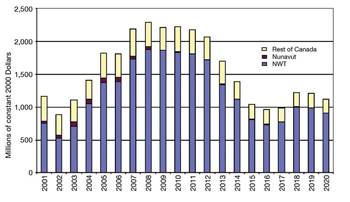
FIGURE 16: Annual contribution of the Tibbitt to Contwoyto Winter Road and associated projects to gross domestic product in the Northwest Territories (NWT), Nunavut and rest of Canada, 2001-2020 (EBA Engineering Consultants, 2001).
Although minor on-ice travel occurs in the Yukon (e.g. Dawson City ice crossing, mine access roads and an occasional ice road to Old Crow), the primary ice-road networks are found in the Northwest Territories and Nunavut, the latter having no long-distance all-season highways. Extensive winter-road networks are also found in the northern parts of several provinces (see Chapters 6 and 7). The longest winter road, the 'Tibbitt to Contwoyto Winter Road' (TCWR), is 600 km long, with 495 km located on frozen lakes (EBA Engineering Consultants Ltd., 2001). It is the main supply road for the Ekati and Diavik diamond mines, the Lupin gold mine (currently inactive), the Snap Lake and Jericho mine developments and several other mineral exploration projects. The TCWR is currently licensed and operated by the Winter Road Joint Venture (WRJV), a private-sector partnership between BHP Billiton, Diavik Diamond Mines and Echo Bay Mines, who share the cost based on use, while other companies using the road pay a tonne/kilometre charge. The TCWR typically operates for two months each year, February and March, at an approximate annual cost of $10 million, with trucks running almost 24 hours/day and convoys leaving at approximately 20 minute intervals. Between 1997 and 2003, it carried up to 8000 truck loads per year, each weighing an average 30 tonnes (t), with the load capacity rising as the ice thickens and increases in bearing strength. The economic importance of the TCWR is projected to continue for many years (Figure 16; EBA Engineering Consultants Ltd., 2001).
The main ice-only road in the Canadian North forms a 150 km winter link between the communities of Inuvik and Tuktoyaktuk, and is constructed and maintained by the Government of the Northwest Territories. Numerous smaller winter roads and ice bridges are found throughout the northern territories, with the Northwest Territories public road system almost doubling in length during the winter (approximately 1400 km in total). Operating windows vary by location and year, but typically extend from November – December until March – April.
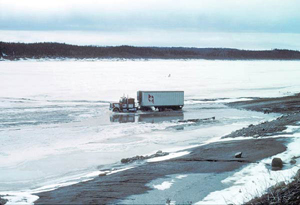
FIGURE 17: Transport truck crossing deteriorating ice road, Liard Ferry crossing near Fort Simpson, Liard River, NT (Terry D. Prowse).
Methods of ice-road construction vary by use, and some also involve tandem development with winter road construction on land. The more formalized road and bridge networks enhance the load-bearing capacity through snow removal or snow compaction to reduce its insulating effects. Rapid ice thickness can be achieved by surface flooding or by spray-ice techniques similar to snow-making. In both cases, the ice thickness can be increased beyond that possible due to normal ice growth for a given set of climatic conditions. Melt events can reduce surface usability even when the load-bearing capacity is high (Figure 17). Large snowfalls near the start of the season can cause a significant delay in ice growth and development of load-bearing capacity.
Although regular records are kept regarding the opening and closing of ice roads/crossings, no comprehensive trend analysis of such dates has been conducted. However, observations for the Mackenzie River Crossing Ice Road show that the average opening date for light traffic has been delayed by more than 3 weeks since 1996, whereas it had remained relatively constant (between December 8 and 19) during the previous 30 years or more (Northern Climate Exchange, 2006). Reductions in ice thickness associated with climate warming reduce the maximum loads that can be safely transported. Initially, modifications in ice-road construction (as described previously) may serve as an effective adaptation. At locations where transport capacity is not already maximized, it is possible to modify transport schedules to concentrate shipping into the core portion of the winter when ice thickness is maximized. A possibility also exists for transporting heavy loads over ice with the assistance of balloons, as suggested for the movement of oilfield equipment in Alaska and the Canadian Arctic (cf. Prentice and Turriff, 2002). This combination of impacts associated with decreased length of the ice-road season and reduced ice thickness translates into increased difficulties in resupplying northern communities and industrial sites during winter months.
Where and when the use of winter roads becomes impractical, there will be a need to provide alternative transportation routes. Where an open-water network is viable, increased use of barge transport might be possible. In land-locked locations, such as those currently serviced by the TCWR, construction of land-based roads is likely the only viable option to carry the enormous total annual loads of freight (see Case Study 1). All-weather roads are significantly more costly than winter roads to build and maintain. Costs vary but, as an example, Dore and Burton (2001) cited costs of at least $85 000 per km for all-weather roads to be constructed in northern Ontario, plus costs of between $64 000 and $150 000 per bridge.
4.6 FORESTRY
Significant areas of the Yukon (22.79 million ha) and the Northwest Territories (33.35 million ha) are covered by boreal forest, together constituting about 13% of Canada's total forest cover. The cultural, spiritual, social and economic well-being of many First Nations is dependent on a healthy forest ecosystem. Gathering of food and the exercise of cultural practices are important uses of forest land in the Yukon and Northwest Territories. Less than 30% of the Yukon forest cover is of a species or size that might support timber-harvesting activities (Government of Yukon, 2006), with the majority of merchantable forests located south of latitude 61 °N. Farther north, Yukon forests are more affected by cold soils, poor drainage and aggressive fire regimes.
The Yukon is moving towards a legislative framework and more regulated practices for forestry activities, including the development of a Yukon Forest Resources Stewardship Act. Responsibilities for forest management are shared by the Government of Yukon, First Nation governments and renewable resource councils. The first strategic forest management plan in the Yukon was adopted in December 2004 for the Champagne and Aishihik Traditional Territory (Case Study 3).
Salvage harvest opportunities are currently driving the forest industry in the Yukon in response to recent expansive forest disturbance. In 2005, more than 300 000 m 3 in timber permits were issued to salvage wood from areas that were burned during the record fire season of 2004 (e.g. Green, 2004). The 2004 fires also created the opportunity for a large mushroom harvest, a lucrative post-fire non-timber forest product. In 2006, a request for proposals for 1 000 000 m 3 in timber permits was issued to salvage wood from the spruce bark beetle infestation in the Champagne and Aishihik Traditional Territory of southwestern Yukon (see Case Study 3). The contribution of harvested wood products to the Yukon economy in 2004 was estimated at a million dollars, and is poised to increase because of the salvage operations. In contrast, the estimated contribution of forest products to the Northwest Territories economy in 2004 was only $70 000 (Table 9).
Canada | Yukon Territory | Northwest Territories | Nunavut | |
---|---|---|---|---|
Profile | ||||
Population1 | 32 100 000 | 31 227 | 42 944 | 29 6583 |
Land area (ha) | 979 100 000 | 48 490 000 | 128 120 000 | 200 600 000 |
Forest and other wooded land | 402 100 000 | 22 790 000 | 33 000 000 | 940 000 |
Parks | 26 500 000 | not available | 13 363 | not available |
Resources | ||||
Ownership2 | ||||
Federal | 16% | 100% | 100% | 100% |
Provincial/Territorial | 77% | 0% | 0% | 0% |
Private | 7% | 0% | 0% | 0% |
Forest type2 | ||||
Softwood | 66% | 79% | 53% | 52% |
Hardwood | 12% | 2% | 47% | 48% |
Mixedwood | 22% | 19% | 0% | 0% |
Potential harvest (m3)3 | 238 800 000 | 238 000 | not applicable | |
Harvest (volume) – industrial roundwood (m3)3 | 193 700 000 | 7 000 | 6 000 | |
Harvest (area) – industrial roundwood (ha)3 | 974 472 | 44 | 31 | |
Area planted (ha)4 | 427 051 | 310 | 112 | |
Area seeded (ha)4 | 18 906 | not available | not available | |
Area defoliated by insects and beetle-killed trees (ha)3 | 19 200 000 | 41 640 | not available | |
Number of fires2 | 6634 | 282 | 297 | |
Area burned (ha)2 | 3 300 000 | 1 800 000 | 515 621 | |
Industry | ||||
Value of exports2 | $44 600 000 000 | $961 842 | $69 954 | |
Softwood lumber | 24.71% | 1.5% | 17.61% | |
Major export markets2 | ||||
United States | 80% | 100% | 37.38% | |
European Union | 6% | 0% | 62.62% | |
Japan | 5% | 0% | 0% | |
Source: Natural Resources Canada (2005); data reported are from Statistics Canada 1 for 2005 2 for 2004 3 for 2003 4 for 2002 |
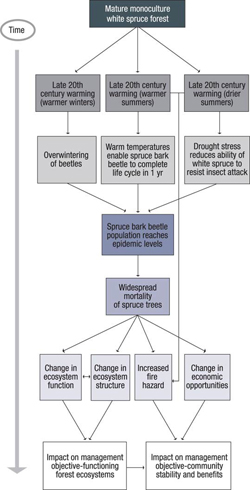
FIGURE 18: Influence of climate warming on spruce bark beetle populations in the southwest Yukon (Ogden, 2006).
The mounting evidence of local ecological responses to recent climate change demonstrates the sensitivity of northern forested ecosystems to climate change (Parmesan and Yohe, 2003; Juday et al., 2005; Ogden, 2006; Scholze et al., 2006). Indeed, many of the projected impacts of changing climate on the northern forest sector (Table 10) are already visible. Increased forest disturbances due to insect outbreaks are almost certain to result from continued climate warming (Juday et al., 2005). The spruce bark beetle infestation of southwestern Yukon, which has led to widespread mortality of white spruce, is the largest and most intense outbreak to affect Canadian trees and is a notable example of ecosystem response to recent warming (Table 11; Figure 18; see Case Study 3; Juday et al., 2005). Climate change is also projected to increase the frequency, extent and severity of forest fires, thereby reducing mean fire return intervals, shifting age class distributions toward younger forests, triggering more frequent shifts from conifer- to deciduous-dominated successional trajectories, and decreasing the amount of terrestrial carbon
stored in the boreal forest (Flannigan et al., 2000; Stocks et al., 2000; Juday et al., 2005; McCoy and Burn, 2005; Johnstone and Chapin, 2006).
Biophysical impact | Socioeconomic impacts |
---|---|
Changes in forest productivity | Changes in timber supply and rent value |
Increased atmospheric greenhouse gases | Introduction of carbon credit-permit mitigation policies that create a carbon sequestration market |
Increased disturbances | Loss of forest stock and non-market goods |
Northward shift of ecozones | Change in land values and land-use options |
Change in climate and ecosystems | Economic restructuring leading to social and individual stresses |
Ecosystem and specialist species changes | Changes in non-market values |
Ecosystem changes | Dislocation of parks and natural areas, increased land-use conflicts |
Direct controls on insect populations: |
|
---|---|
Indirect control on tree resistance: |
|
'Fingerprint' of climate warming: |
|
CASE STUDY 3
Building the Foundation for Forest Management in a Changing Climate
There is accumulating evidence that climate change is affecting the Champagne and Aishihik Traditional Territory of the southwestern Yukon. During the past 40 years, average annual temperatures have been increasing and winters have had fewer periods of prolonged severe cold. There has been a decrease in average summer precipitation. One result of these warmer winters and warmer and drier summers has been a severe outbreak of spruce bark beetle (Dendroctonus rufipennis), causing widespread mortality of white spruce. This mortality has also led to the loss of merchantable timber and significant changes to the regional ecology.
The spruce beetle infestation has also increased quantity, flammability and extent of forest fuels, thereby increasing the fire hazard. The spruce bark beetle outbreak is driving forest management and planning efforts in the southwestern Yukon. In November 2004, the Government of Yukon and the Champagne and Aishihik First Nation approved the first community-directed forest management plan that identifies reduction of fire hazard, forest renewal, economic benefits and preservation of wildlife habitat as forest management and planning priorities. The plan also explicitly incorporates an adaptive management framework, considered to be an essential response to climate change. Examination of forest management actions that could be undertaken to reduce the vulnerability of forest ecosystems, and the people and economies that depend on them, to climate change was undertaken by the Northern Climate Exchange (Ogden, 2006). Activities included a workshop on 'Our Changing Boreal Forest', hosted by the Champagne and Aishihik First Nation and the Alsek Renewable Resource Council, and involving local residents, governments and management agencies, and researchers. The workshop outcomes provided a foundation for a preliminary research framework to support forest management decision-making in the changing climate of southwestern Yukon.
The region has been designated a Special Project Area of the Canadian Model Forest Program, securing funding for the Champagne and Aishihik First Nation to do additional research on issues of community sustainability. Future work will include seeking the perspective of community members on the applicability of various adaptation management actions in the local context, conducting a scenario planning exercise to examine the effectiveness of forest management options under possible future climates, and incorporating traditional and localknowledge into the adaptive management framework.
Depending on species, site type and region, warmer temperatures in the last several decades have either improved or decreased tree growth. In some areas where declines have been observed, drought stress has been identified as the cause, whereas declines in other areas remain unexplained (Juday et al., 2005). Drought stress is also impeding the re-establishment of spruce forests following fire in some areas of southwestern and south-central Yukon, which are highly vulnerable to climate change if trends towards drier conditions continue (Hogg and Wein, 2005). Most projections of future climate result in conditions that are very likely to limit the growth of commercially valuable white spruce types and widespread black spruce types in large parts of Alaska and probably the western boreal forest of Canada (Barber et al., 2000; Hogg and Wein, 2005; Juday and Barber 2005; Juday et al., 2005). Climate-related changes in forest productivity will likely have significant impacts on northern forest-dependent communities (Hauer et al., 2001; Davidson et al., 2003). The principles and practice of sustainable forest management embody many of the activities that will be required to respond to the effects of climate change (Spittlehouse and Stewart, 2003). Of those forestry practitioners in the Yukon and Northwest Territories who were surveyed about the likely impacts of climate change on forest sector sustainability and potential adaptation options, 71% agreed that the seven criteria of sustainable forest management3 could also serve as goals for climate change adaptation in the forest sector (Ogden and Innes, in 2007b). The three impacts most frequently identified as already having affected sustainability were changes in the intensity, severity or magnitude of forest insect outbreaks; changes in extreme weather events; and changes in the intensity, severity or magnitude of forest fires (Table 12; Ogden and Innes, in 2007b). However, more than half of the respondents indicated that commodity prices, availability of timber, trade policies, environmental regulations and the ability to secure needed capital presently have more of a negative impact on sustainability than climate change (Table 13; Ogden and Innes, in 2007b).
Climate change impact | Respondents (%) |
---|---|
Intensity, severity or magnitude of forest insect outbreaks | 66 |
Extreme weather events | 47 |
Intensity, severity or magnitude of forest fires | 44 |
Lifestyles | 34 |
Land values and land-use options | 31 |
Length of winter road season | 31 |
Economic opportunities | 25 |
Forest carbon budget | 22 |
Phenology | 22 |
Timber supply | 22 |
Species abundance, movement and ranges, including invasive species | 19 |
Forest cover type | 19 |
Forest growth and productivity | 16 |
Location of treeline | 16 |
Availability of non-timber forest products | 9 |
Influence on sustainability is presently more important than that of climate change | Respondents (%) |
---|---|
Commodity prices | 56 |
Availability of timber | 53 |
Trade policies | 50 |
Environmental regulations | 50 |
Ability to secure needed capital | 50 |
Competitiveness | 47 |
Oil and gas | 41 |
Habitat fragmentation | 41 |
Aboriginal involvement and governance | 35 |
Public participation in forest management and planning | 35 |
Minerals | 35 |
Tourism | 35 |
Community health and well-being | 35 |
Invasive species | 29 |
Unemployment | 29 |
Contaminants | 26 |
Participation in traditional lifestyles | 21 |
Availability and security of traditional food supplies | 18 |
Availability of recreational opportunities | 15 |
Ozone depletion | 6 |
Despite not perceiving climate change as the most important influence on forest sector sustainability (Ogden and Innes, 2007b), northern forest managers are already adapting reactively to the impacts of changing climate, most notably in response to the spruce bark beetle infestation in southwestern Yukon (see Case Study 3; Alsek Renewable Resource Council, 2004). Possible proactive adaptations include targeted regeneration, silviculture or protection strategies to address long-term shifts in forest disturbance patterns (Ohlson et al., 2005). Proactive adaptation is more likely to avoid or reduce damage than reactive responses (Easterling et al., 2004). The perspectives of forest practitioners on the importance of adaptation options that may be considered to meet the goals of sustainable forest management were explored. The options assessed are presented in Table 14 (Ogden and Innes, 2007b). Practitioners also identified what they felt were the most important research needs to assist decision-making. These include understanding the impacts of climate change on the intensity, severity and magnitude of forest insect outbreaks and forest fires, as well as net impacts on forest growth and productivity (Ogden and Innes, 2007b).
Adaptation goals | Conserve biological diversity | Maintain productive capacity of forest ecosystems | Maintain forest ecosystem health and vitality | Conserve and maintain soil and water resources |
---|---|---|---|---|
Strategic-level adaptation options |
|
|
|
|
Operational-level adaptation options |
|
|
|
|
Adaptation goals | Maintain forest contribution to global carbon cycles | Maintain and enhance long-term | Multiple socioeconomic benefits | |
Strategic-level adaptation options |
|
|
|
|
Operational-level adaptation options |
|
|
||
Sources listed in Ogden and Innes, 2007a. |
4.7 FISHERIES
The northern fish fauna of Canada consist of an estimated 240 species (190 marine, approx. 15 anadromous, and approx. 35 freshwater forms; Richardson et al., 2001; Evans et al., 2002; Coad and Reist, 2004). Additional fish species, not yet recorded due to poor sampling coverage, likely also occur in northern, particularly marine, waters. Adjacent regions contain additional species that may eventually be found in the North. Of the endemic species, northern fisheries target relatively few (approx. 11 species), most of which are salmonids (e.g. salmon, chars, whitefishes, grayling) captured in fresh, estuarine or near shore waters. About five freshwater species are targeted (e.g. burbot, northern pike, suckers and perches). A further limited number (2–3) of marine fish species (e.g. Greenland halibut, Arctic cod) and a few (3–6) invertebrate species (e.g. shrimps, clams, mussels and urchins) complete the suite of exploited taxa (Nunavut Wildlife Management Board, 2004; Government of Nunavut and Nunavut Tuungavik Incorporated, 2005; Reist et al., 2006a). Some additional species may be fished locally and/or captured as by-catch in fisheries and either discarded or used for bait or dog food.
The number of species present in the region is likely to rise as climate changes, especially along the southern margin of the North. Several southern species are known to occur as vagrants in the North, including three species of Pacific salmon in the western Arctic and Atlantic salmon in the east. Colonization could result in new opportunities for fisheries, but could also add to existing stressors as ecosystems restructure, new predators appear, competition ensues and/or parasites are introduced by the colonizing species (Reist et al., 2006b, c; Wrona et al., 2006a). Experience with the vagrants in local fisheries enhances interest in future potential for fisheries based upon those species.
Freshwater and anadromous species can be divided into three groups, based on thermal associations and preferences (see Wrona et al., 2005; Reist et al., 2006a):
- Arctic (thermal tolerance <10°C): species that are wholly or primarily distributed in the north (e.g. broad whitefish, an anadromous fish of the western Arctic)
- northern cold-water–adapted (11–15°C): species that reach their limits of distribution somewhere in the North
- southern cool-water–adapted (21–25°C): many of these species (e.g. Atlantic salmon) reach the northern limit of their distribution near the extreme southern margin of the North
Changing climate will affect these three groups, and associated fisheries, differently. Arctic species will likely experience declining productivity, local extirpation along the southern margin of their distribution and overall range contraction as local conditions exceed thresholds and southern species colonize and compete with or prey upon them. Both northern cold-water and southern cool-water species will likely increase in abundance and local productivity, and perhaps also extend their geographic range farther northward as conditions allow.
Particular fish species are either stenothermal (i.e. adapted to a narrow range of temperatures) or eurythermal (i.e. adapted to wide thermal ranges; e.g. Wrona et al., 2005; Reist et al., 2006a). These species are often captured together in the same fishery. In many cases throughout the North, local climate change may be positive for one species and negative for another. Such variability in the response will substantially affect fishery structure, output and sustainability, and present challenges to those fishery managers who rely primarily upon single-species management approaches. Management structures and approaches that focus on the ecosystem level are likely to be more highly responsive to climate change impacts. An ecosystem approach involves attaching differential values to local species and enabling the setting of attainable goals for sustainable fisheries and their management.
Northern fisheries can be classified into three types, household, commercial and recreational, based upon the final disposition of the catch (Clarke, 1993). Household fisheries include traditional and subsistence food fisheries conducted by Aboriginal people, as well as licensed domestic fisheries conducted by non-Aboriginal northerners. Commercial fisheries are licensed activities in which the product is sold either locally or in distant markets. Recreational or sport fisheries are licensed individual fisheries by non-Aboriginal persons. Each type of fishery and area will be affected differently by climate change, making the development of generalizations that are applicable to fisheries throughout the North difficult. Although detailed assessment of all northern fisheries is beyond the scope of this chapter, many of the issues and challenges are developed further in Case Study 4.
Commercial fisheries (see Case Study 4), and the very limited aquaculture occurring in the North, are generally small and widely dispersed, and conducted on small water bodies by local residents for both food and income. This results in limited economic potential measured in such typical terms as commercial cash income; however, valuation of these numerous widely dispersed fisheries must also include estimates of protein replacement and social and cultural value. In Nunavut, fisheries are estimated to contribute between $12 and 14 million annually to the economy (Government of Nunavut and Nunavut Tuungavik Incorporated, 2005). Of this, $5.8 million is estimated to accrue from Arctic char, of which $1.4 million comes from commercial sales of 800 –1000 t annually and $4.4 million from the food value for subsistence use.
Inshore coastal marine and lake-based commercial fisheries and aquaculture operations are likely to face significant adaptation challenges as a result of changing climate. In addition to fairly intensive capitalization of the fishing fleet, these fisheries are supported by harbour facilities and onshore fish-processing facilities that require significant capital expenditures and regular inspection and maintenance to maintain standards for processing commercial fish products. In the North, long-term, relatively stable production is required to recoup initial investments. Current views of such activities in the North being a major contributor to economic development in the future (e.g. Government of Nunavut and Nunavut Tuungavik Incorporated, 2005) may have to be adjusted in view of the consequences of changing climate.
Inherent adaptive ways of life and resiliency of northern Aboriginal peoples will aid the process of adjusting traditional and subsistence fisheries to changing climate. By their nature, recreational fisheries are highly adaptable with respect to harvest levels, gear used and location of fishing. Thus, sport fisheries will likely be readily able to accommodate the impacts of climate change, the possible exception being where there is widespread loss of a species over a large area, in which case reprofiling such fisheries to 'new' species might be possible.
CASE STUDY 4
Commercial and Subsistence Arctic Fisheries
This case study describes and contrasts three different northern fisheries to illustrate the challenges that changing climate presents to resource management.
Nunavut Commercial Fisheries on Greenland Halibut
Both inshore and offshore commercial fisheries for Greenland halibut have been developed in Nunavut. Greenland halibut is a flatfish typically found near the bottom in deep waters of Baffin Bay and Davis Strait, as well as inshore in deeper fjords.

FIGURE 19: Hauling long-lines; inshore ice fishery. Photograph courtesy of Nunavut Government.
The inshore fishery is typically conducted through landfast ice on Cumberland Sound from December to March (Figure 19), with a quota presently set at 500 t. This fishery has been operating since 1987, with harvests varying from 4 to 430 t, 6 to 115 fishers involved annually and a season of 9 to 21 weeks duration. Recent high variability in sea-ice formation has affected the ease of travel and safety of fishers accessing the fishing grounds. In some years, ice formation has been quite distant from the best locations, resulting in low success of the fishery. This discourages entry and continued participation in the fishery, which in turn causes decreased employment at the processing plant and decreased local economic benefit.
The projected impacts of climate change on sea-ice conditions will have a significant effect on this inshore fishery. One possible adaptive response would be to diversify the inshore fishery to encompass a wider resource base, thereby increasing the resilience of the community to perturbation from climate or other factors. More extensive use of the existing Exploratory Fisheries Program could foster such diversification. The economic, social and societal benefits of diverse local fisheries have been documented for Greenland and other North Atlantic fishing communities (e.g. Hamilton and Otterstad, 1998; Hamilton et al., 2000).
The offshore fisheries in this area are also significant, accounting for 550 t of the total Canadian quota. It is a deep-water, bottom-trawl fishery involving large vessels during open-water season. Although subject to minor interannual variability due to seasonal ice conditions, access to the fishing grounds will be either unaffected or improved as a result of changing climate, although ice hazard risks may be similar to or greater than those at present. Little is known about how shifts in freshwater budgets will affect Greenland halibut production. Loeng et al. (2005) indicated that substantive restructuring of marine ecosystems will occur under changing climate, with Greenland halibut likely moving from deeper waters to shelf areas, affecting where and how fisheries could be conducted. This will necessitate adaptation (e.g. shift in gear type and possibly in vessel size) on the part of the existing fishery fleet.
Great Slave Lake Fisheries, Northwest Territories

FIGURE 20: Lifting whitefish nets on Great Slave Lake. Photo courtesy of George Low (Fisheries and Oceans Canada).
Great Slave Lake, the eleventh largest lake in the world, supports commercial fisheries with an annual recent catch of approximately 1200 t. The principal species fished are lake whitefish, northern pike and inconnu (Figure 20). The East Arm of Great Slave Lake supports trophy sport fisheries, primarily for lake trout. Household fisheries occur in nearshore areas and local tributaries. All fishery types target multiple species. To minimize conflicts and maximize conservation and the value and sustainability of the various fisheries, fisheries managers use a system of area closures, quota limits and gear restrictions to limit both commercial and recreational activities. These same actions will likely be important tools for dealing with the impacts of changing climate.
Projected impacts of changing climate include a potential 50% increase in the number of 'optimal growing season' days for cold-water fish, such as lake trout, in the East Arm (McLain et al., 1994). In the relatively shallow west basin of Great Slave Lake, climate-related changes are likely to stress lake trout populations, but species with higher upper thermal tolerances, such as lake whitefish, will likely be positively impacted in terms of increased growth. Structural shifts in the lake ecosystem will likely occur as more southerly species from Alberta river systems, which are currently limited by climate, colonize and/or increase in abundance in the lake. Individual and cumulative effects of these impacts cannot be estimated with confidence because of a lack of baseline information. Adaptive management of the lake ecosystem needs to be done in the context of cumulative effects, including non-climate stresses.
Arctic Char Subsistence Fisheries
Traditional and subsistence fisheries for Arctic char and related char species are conducted wherever these fish occur, particularly in the coastal western Arctic and throughout Nunavut. Data from the harvest study conducted by the Nunavut Wildlife Management Board (2004) indicate that this species constitutes 45% (by number caught) of the top 15 harvested species reported from 1996–2001 (Figure 21). Other estimates indicate annual harvests of 1200–1500 t (Government of Nunavut and Nunavut Tuungavik Incorporated, 2005), with a high value to local economies and ways of life. All Nunavut, and most Inuvialuit and Gwich'in communities report harvests of either Arctic char or Dolly Varden. Traditional subsistence fisheries have tended to operate in a conservative fashion and are small, widely dispersed and usually short term, with low overall impact. Increases in Aboriginal populations and centralization in settlements, however, have recently limited the efficacy of this approach to northern subsistence fisheries. Where char are exploited by multiple fisheries (subsistence/household, commercial and recreational), current northern fishery management ranks traditional and subsistence fisheries as the most valued (Clarke, 1993).

FIGURE 21: The frequency of Arctic char reported as harvested in subsistence fisheries in Nunavut as overall counts of the top 15 harvested organisms reported in the Nunavut Harvest Study from 1996-2001 (Nunavut Wildlife Management Board, 2004). Figure compiled by B. Dempson, Fisheries and Oceans Canada.
Projected effects of changing climate on char will involve shiftsin productivity and biodiversity, including changes from predominantly anadromous to resident life histories, complete extirpation in some areas and local declines in abundance in other areas (Wrona et al., 2005; Reist et al., 2006a). These shifts in the biological base of the fishery will have cascading ramifications. They will necessitate a variety of local adaptive responses on the part of the fishers and fishery managers, including shifting places, times or methods of capture; switching to alternative species (or life history types); adjusting levels of exploitation; and, in some cases, altering expectations and value associated with fishery resources (Reist et al., 2006c). Another key consideration is the interaction of climate change with contaminant dynamics that affect fish productivity and quality (Wrona et al., 2005), and hence fish suitability for human consumption. Monitoring of such effects, and of human health impacts, should be part of general approaches to northern fisheries.
An effective generalized approach to climate change adaptation involves comprehensive management and understanding of all human activities that impact northern aquatic ecosystems. For pervasive impacts in the North, such as contaminant loading, climate change, ozone depletion and increased incident ultraviolet radiation, virtually no quantification of the effects on fish populations is available. As a result, adaptive strategies should incorporate a wide buffer to enhance resiliency in the system. For fisheries, this likely means revising 'sustainable' strategies to levels below what is assumed or known to represent the 'maximum sustainable level' or 'total allowable harvest', as this most often will be the only factor that can be managed. Priority might be placed on ecosystems undergoing, or projected to undergo, the greatest changes from all stressors. It presently appears that aquatic systems in the western Arctic, particularly in the southern portion of the Yukon, along the Mackenzie Valley to the delta and along the Beaufort Sea coast, are undergoing significant shifts (e.g. Prowse et al., 2006) and are therefore at greatest overall risk.
4.8 WILDLIFE, BIODIVERSITY AND PROTECTED AREAS
A diverse range of wildlife has been critically important for Aboriginal people in the Canadian North for thousands of years. Today, wildlife continues to play a vital role in the diet, traditions and cultures of Aboriginal people, and also forms important components of local and regional economies (Nuttall et al., 2005). Many Arctic terrestrial and marine mammals and bird species have narrow habitat and niche requirements that make them particularly sensitive to climate change (Conservation of Arctic Flora and Fauna, 2001). Range-restricted wildlife species that occur near their ecological limit have been some of the first to exhibit impacts from changing climate (Parmesan, 2006). Previous assessments of projected climate change on circumpolar Arctic wildlife highlight changes in mortality rates, reduced reproductive capacity, increased competition for resources due to northward extension of southern species, and the emergence of new zoonotic diseases (Berner et al., 2005; Chapin et al., 2006).
Such changes will impact key traditional, subsistence and economic species in some regions, and their effects could be reduced by means of proactive adaptation to reduce the implications for human populations relying on these resources.
Terrestrial Species
Projected warming and shifts towards a wetter Arctic are expected to affect the diversity and accessibility of vegetation critical to several foraging mammals, such as woodland and barren ground caribou (Rangifer tarandus) and muskox (Ovibos moschatus). Changes in ultraviolet radiation, precipitation and temperature will directly affect the nutritive content of forage (Lenart et al., 2002), while changes in the composition of flora communities may result in the loss of nutritionally important plant species, which are chosen by caribou during important reproductive stages (White and Trudell, 1980). Projected increases in winter temperature and precipitation will likely result in an increase in energy expended by caribou to dig for food through deeper snow pack (Russell et al., 1993).
Some barren ground caribou in the western and central Arctic, including the Bluenose East and West, Cape Bathurst and Porcupine herds, have experienced significant declines in recent years, at least partly attributable to changes in climate. Declines have been associated with difficulties in obtaining appropriate forage and increased harassment by insects that interrupts summer feeding, resulting in poorer animal condition and a subsequent reduction in reproduction and recruitment (Russell, 1993). Similarly, recent declines of caribou and muskox occupying the islands of the south-central Arctic Archipelago (Prince of Wales, Somerset and Russell) are partly attributable to large-scale winter die-offs of both species associated with decreased access to forage due to harsh winter weather, including heavy snow events and increased icing (Miller and Gunn, 2003; Harding, 2004; Gunn et al., 2006; Tesar, 2007). Similar conditions on the western islands of the high Arctic are reported to be the cause of decline among Peary caribou, currently listed as a 'threatened' species. Additional factors involved in the decline of some herds include increased competition between caribou and muskox occupying the same habitat, increased incidence of parasitic infection, emigration to adjacent areas, wolf predation and hunting (Gunn et al., 2006). Similar impacts were projected by Brotton and Wall (1997) for the Bathurst caribou herd using four future climate scenarios.
Caribou are a key traditional and subsistence species for Aboriginal peoples of the Canadian Arctic, including Gwich'in, Tli cho, Denesuline and Inuit, and play an important role in local nutrition, economies, cultures and spirituality. The climate sensitivity of caribou highlights the need to monitor and better understand changes in small and genetically unique groups of animals, and to adjust wildlife management strategies accordingly (Miller et al., 2005; Gunn et al., 2006). Adaptation measures will need to limit the chance of undetected large-scale herd die-offs and harvesting above sustainable levels, in order to protect species from declines to levels from which they cannot recover (Brotton and Wall, 1997; Klein et al., 2005). Wildlife management boards in the Northwest Territories are currently considering implementing measures to reduce the non-resident and non-Aboriginal harvest of caribou (Tesar, 2007). Adaptive co-management strategies, involving local Aboriginal harvesters and bringing together scientific and traditional knowledge, are becoming increasingly important (Klein et al., 2005; Parlee et al., 2005).
Marine Species
The Arctic marine environment is home to a variety of large mammal species that have adapted to the unique conditions of this ecosystem. Ringed sea (Phoca hispida), walrus (Odobenus rosmarus), narwhal (Monodon monocerus), polar bear (Ursus maritimus) and beluga whale (Delphinapterus leucas) are widely found throughout Northern Canada and are commonly harvested by coastal Aboriginal populations (Nuttall et al., 2005), conveying important health, economic and cultural benefits (Van Oostdam et al., 2005). Many of these species are also central to important Arctic tourism and sport-hunting ventures. Changes in the distribution, stability and annual duration of sea ice and snow availability will have significant impacts on the populations of these mammals. Some species dependent on sea ice as a suitable platform for resting, pupping, moulting or feeding are already showing stress at their southern limits (Learmonth et al., 2006). Species that rely on the ice-edge environment, such as beluga, narwhal and walrus, are most vulnerable to the effects of projected decreases in sea-ice cover (Learmonth et al., 2006).
Decreased snow depth and earlier ice break-up in the spring have been shown to affect the survival and recruitment of ringed seal (Phoca hispida) pups in western Hudson Bay, while early spring warming and rainfall have been linked to the melting and destruction of ringed seal lairs in southeastern Baffin Island (Stirling and Smith, 2004; Ferguson et al., 2005). A review of changes in sea-ice conditions and suitability of habitat for ringed seals in M'Clintock Channel and the Gulf of Boothia by Barber and Iacozza (2004) showed large interannual variability, yet negative impacts on ringed seal habitat were evident between 1997 and 2001. For some other seal species, including harbour seals and grey seals, climate warming and decreases in sea-ice cover will mean a northward expansion of their distributions and an increase in their prevalence in Arctic waters.
The distribution of polar bears (Ursus maritimus) is partly a function of the ice conditions that allow them to most efficiently hunt and travel. This relationship is particularly strong in areas of moving ice, between foraging grounds and where they give birth to and rear their young (Learmonth et al., 2006). As polar bears feed almost exclusively on ringed seals, changes in ice distribution and extent that impact seal populations will also affect polar bear distribution and foraging success. A significant decline in the numbers and condition of southern populations of adult bears has been documented in western Hudson Bay, and is associated with changes in sea-ice conditions and seal populations (Stirling et al., 1999; Ferguson et al., 2005). Such changes require bears to travel longer distances in search of seals, and to diversify their diet when possible, expending more energy and depleting adipose stores. Ultimately, this can lead to reproductive impairment in females and decreased health of cubs, as mothers have less fat stores during winter months (Derocher et al., 2004). Changes in the proportions of different seal species in the diet of polar bears in Hudson Bay are further evidence of the cascading effects that changing climate and other stressors are likely having on bears in this region (Stirling, 2005). It has also been suggested that changes in ice conditions, as well as specific intraspecies and interspecies competition, have resulted in some bear mortalities in the Beaufort Sea (Amstrup et al., 2006; Monnett and Gleason, 2006).
Projected climate change is likely to lead to improved habitat conditions for both seals and bears at higher latitudes in the near future, as multi-year ice is replaced with annual ice and more leads and pressure ridges are created. In the longer term, however, it is likely that impacts similar to those currently seen in Hudson Bay will also be experienced in high-latitude areas (Derocher et al., 2004).
The iconic nature of the polar bear as a symbol of the Canadian North has elevated discussion of its fate. Not only are polar bears an important component of the Arctic ecosystem, but they are also a key attraction for many visitors to the North each year, and play a significant role in the culture and economies of many Aboriginal communities. Their economic value in regions where high success is achieved in sport hunts, such as western Hudson Bay and Lancaster Sound, is significant for Nunavut communities, where a tag alone can attract as much as $20 000 from a non-resident hunter, with additional income obtained from guiding and outfitting fees garnered by residents in the community (Wenzel, 2005; Freeman and Wenzel, 2006). Thus, the impacts on bears of shifting climate regimes also have implications for tourism, culture and local economies in many regions.
The potential effects of climate change on Arctic whale species are less well understood than for other large marine mammals (Loeng et al., 2005). It is expected that changes in the distribution and extent of ice cover, and the formation and location of polynyas will influence prey species, and thus affect the distribution and range of many northern cetaceans. In general, warming will cause shifts in species composition, with a tendency for northward movement in community structures and the potential loss of some polar species (Tynan and DeMaster, 1997). For example, Moshenko et al. (2003) ranked climate change as a 'high' threat to bowhead whales (Balaena mysticetus).
Bird Species
Many sea birds and other migratory avian species are consumed by Aboriginal residents of the North and provide local-scale economic resources in some regions (e.g. eider down). Warming waters and changes in ice distribution and prey productivity are already impacting some northern bird species. The ivory gull (Pagophila eburnea), whose distribution is linked to sea ice, has undergone population reductions and further reductions are projected in the future (Mallory et al., 2003). Studies of Brunnich's guillemot (Uria lomvia) have shown changes in the timing of breeding at both the northern and southern limits of its range and an advancement of egg-laying dates, yet lower chick growth rates and adult body mass at its southern limits and reduced reproductive success in years of late freeze-up in the north. Gilchrist and Robertson (2000) showed the importance of small recurring polynyas and ice floe edges as wintering habitat for species such as old squaw (Clangula hyemalus) and king eider ducks (Somateria spectabilis) in Hudson Bay. Throughout the North, Aboriginal hunters have reported changes in migration routes, timing, breeding and reproductive behaviour of birds, as well as the appearance of previously unseen species (e.g. Huntington et al., 2005; Nuttall et al., 2005; Nickels et al., 2006). Together, these results show that warming in the Canadian Arctic in the near future should continue to positively affect some bird species at their northern limits, while having negative impacts at their southern extremes (Gaston et al., 2005).
Conservation and protection of migratory bird resources in the face of changing climate will be challenging in the Arctic, particularly in areas where industrial activity, tourism and increases in local human population provide additional stressors. Protecting key marine areas could play an important role in maintaining ecosystem integrity and thus protecting sea bird populations (Dickson and Gilchrist, 2002). Management strategies involving resource users (Aboriginal residents and others) and industry representatives will have to consider the many stresses on bird populations.
Contaminants and Wildlife
Climate-related changes that have already been observed in the North also influence wildlife exposure to, and intake of, environmental contaminants (Macdonald, 2005; Macdonald et al., 2005). Many environmental contaminants are transported towards polar regions via air and water currents, and changing climate is altering these contaminant pathways (Arctic Monitoring and Assessment Programme, 2003b). For the metals lead, cadmium and zinc, the Arctic is likely to become a more effective 'sink' because of projected increases in precipitation. Mercury appears to be increasing in some northern aquatic systems, related partly to changes in ice cover and permafrost melting. Migratory species represent a form of biotransport, changing the distribution of contaminants they contain. Areas seeing the appearance of new species are most vulnerable to negative changes in contaminant loads in the future. Finally, hydrocarbons will be affected by changes in sea-ice distribution and drift tracks (Macdonald et al., 2005). The largest change in contaminant movement into or within the Arctic may occur as the Arctic Ocean becomes increasingly open to transportation, tourism and mineral exploration.
Changes in contaminant composition and levels in key wildlife species that are consumed by northern residents are significant for human heath and well-being (Kraemer et al., 2005; see Section 5). To more accurately track trends of contaminants in key wildlife species in the future, and ascertain the effects of climate on their levels, there is a need to concurrently collect data in both biotic and abiotic media (Macdonald et al., 2005).
Biodiversity and Protected Areas
Climate change is expected to affect Arctic biodiversity through changes in the distribution of ranges and habitats of species, the abundance of species, the genetic diversity and behaviour of migratory species, and the introduction of non-native species (Usher et al., 2005). Current plans for parks and protected areas adopt a natural region or ecoregion representation approach, designed to protect specific natural features, species and communities of a site. These plans generally do not consider the landscape-level shifts in ecosystem distribution and structure that are likely to result from changing climate (Lemieux and Scott, 2005).
Using two global vegetation models and a number of climate models, Lemieux and Scott (2005) projected a decline of more than 50% in conservation lands from each of the three northern biomes (tundra, taiga/tundra, boreal) under a scenario of doubled atmospheric concentrations of carbon dioxide. Such projections raise concerns about the adequacy of existing plans to continue protecting representative samples of Canadian Arctic ecosystems, and ultimately Arctic biodiversity. Usher et al. (2005) concluded that there is an immediate need to develop and adopt new approaches to managing Arctic biodiversity. Adaptive conservation and protected area plans should consider projected changes in phenology and the movements of individual species in response to changing climate, as well as potential changes in biological communities. Disruption of competitive or predator-prey interactions could jeopardize sustainability of ecosystem services on which humans rely (e.g. Root and Schneider 1993;
Millennium Ecosystem Assessment, 2005). This is particularly important for species in areas projected to incur cumulative stresses related to climate change, increased development and other human activities.
4.9 TOURISM
The Arctic has seen increased interest and visitation by tourists in recent years (Stewart et al., 2005). Although standardized tourism statistics are lacking for the region as a whole (Pagnan, 2003), some indicators of the level of visitation and the importance of these visitors to local and regional economies are available. The Yukon sees the greatest economic benefits from tourism, with approximately 32 000 tourists in 2002 generating approximately $164 million in economic value (Pagan, 2003). Although hunting and fishing visitors account for only 14% of all visits in the Northwest Territories, they generate approximately 45% of annual spending by tourists in the territory. Tourism is the fourth largest economic sector in Nunavut, with 18 000 visitors entering Canada's newest territory in 2003.
Economic development has long been the driving force behind tourism in the North (Stewart et al., 2005). Although there are obvious benefits to economic diversification, there are also concerns regarding the impacts of tourism on northern communities and local businesses. Inuit in Clyde River, NU, for example, have expressed interest in opportunities associated with tourism as long as the development of these activities is gradual and the community maintains control (Nickels et al., 1991; Stewart et al., 2005).
Challenges facing the tourism industry in the Arctic include a short travel season, transportation difficulties, costs of infrastructure and dependence on nature. Potential positive impacts of changing climate for the tourism industry are associated with increased access and longer travel seasons. An increasingly navigable Northwest Passage could increase tourism opportunities for cruise ships through this relatively unseen wilderness (Stewart et al., 2005), although ice hazards are likely to remain high for several decades (see Sections 3.1 and 4.5.1).
Implications for Economic Development and Adaptation within Key Sectors
Page details
- Date modified: