4 NEW ENERGY EFFICIENT TECHNOLOGIES THAT ARE APPLICABLE TO MANUFACTURING PROCESSES
In recent years, many technological innovations have enabled the processes in the food and beverage industry to become more efficient, less cumbersome, safer, less energy intensive and more environmentally friendly.
Several proven techniques and processes for separation, thermal treatment, bacterial control and energy reclamation are presented in this section. Since the purpose of this guide is to point the reader toward solutions that are applicable in an industrial context, we have chosen to limit ourselves to techniques that are well proven and available on the market. These technologies are illustrated with practical applications that demonstrate their energy efficiency in the meat, beverage and dairy processing sectors.
Note that several other techniques, which are still being developed are expected on the market in a few years.
4.1 Membrane filtration
Membrane filtration is used to remove particles that are too fine for ordinary filtration techniques – such as proteins, bacteria, viruses and dissolved salts – from liquids. It can also be used for concentrating, fractionating, purifying and regenerating liquids, partially or totally replacing traditional methods of separation by evaporation and centrifugation.
Figure 4-1 – Separation capacity of various membrane filtration technologies
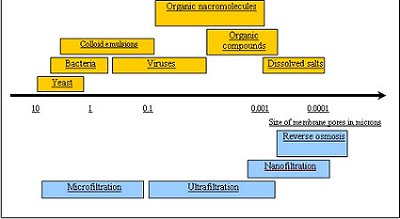
Text Version
An arrow extends horizontally across the centre of the image. Along the arrow appears the “Size of membrane pores in microns” in increments, as follows: 10, 1, 0.1, 0.001, 0.0001. Above the arrow appear yellow rectangles containing the following labels: Yeast (10), Bacteria (1), Colloid emulsions (1 to 0.1), Viruses (0.1), Organic nacromolecules (0.1 to 0.001), Organic compounds (0.001), Dissolved salts (0.0001). Below the arrow appear blue rectangles containing the following labels: Microfiltration (10 to 0.1), Ultrafiltration (0.1 to 0.001), Nanofiltration (0.001 to 0.0001) and Reverse osmosis (0.0001).
In the agroprocessing industry, we are likely to encounter four types of membrane technologies, depending on the application: microfiltration, ultrafiltration, nanofiltration and reverse osmosis (RO). These methods are differentiated by their separation capacity, which is a function of the membrane pore size, and the molecular mass of the particles we want to remove.
Although these technologies have already proven their worth in several industrial applications, there remains ample room for growth.
In many cases, they can partially or totally replace other technologies and reduce energy consumption.
At the process entry point |
Treatment of the boiler’s make-up water Preparation and bacterial control of process water Standardizing milk |
Beverages, beer, milk, meats Beverages, beer, milk, meats Milk |
---|---|---|
During the process |
Clarifying juices, beverages, beer Concentrating juices Removing alcohol Standardizing milk Pre-concentrating milk Demineralising whey Eliminating microorganisms and bacteria Reclaiming proteins |
Beverages, beer Beverages Beer Milk Milk Milk Milk, beer, beverages Milk, meats |
At the process exit point |
Regenerating and recycling cleaning solutions (water and reactants) Treating wastewater and recycling water and reactants |
Milk, beer, meats
Beverages, beer, milk, meats |
The principal advantages of membrane technologies are as follows:
- a pronounced reduction in energy consumption compared to traditional thermal processes
- proven deployment in several industrial sectors, especially dairy
- environmental benefits arising from increased potential for recycling and reduction or elimination of the use of certain chemicals
Examples of industrial applications
Various industrial applications of membrane filtration are illustrated in the following examples.
Membrane filtration – Beverage industry
Type of business: fruit processing establishment in the United States
Application: production of fruit juice concentrates
Deployment period: from 1990 to 1999, 14 sites
Economic data: not available
Results
– thermal energy required for evaporation: 1.162 megajoules (MJ)/kilogram (kg) of water evaporated
– electrical energy required for membrane filtration: 0.232 MJ/kg (0.065 kWh/kg)
– an 80 percent reduction in energy requirements, corresponding to a 37 percent reduction of the energy bill ($4/gigajoule for natural gas, $0.06/kWh for electricity and 75 percent efficiency in steam generation)
Methodology
A preliminary concentration of the fruit juices with an ultrafiltration module, followed by an RO module in which the membranes selectively separate the water from the other constituents of the juices. The concentration then continues in an evaporator.
Project delivered
Concentrating fresh fruit juices occurs in two stages:
– From the initial 5 percent to 10 percent total dry matter: The operation eliminates 50 L of water per 100 L of fresh juice by using a membrane procedure combining ultrafiltration with RO, resulting in a less energy-intensive process than evaporation.
– From 10 percent to the final concentration, which is between 40 and 62 percent total dry matter, depending on the precise nature of the fruit juice: Next, the juice is concentrated with an evaporator.
Membrane filtration – Dairy processing industry
Type of business: dairy processing establishment in Canada
Application: concentration of cheese whey
Deployment date: 1990
Costs associated with membrane filtration: $400,000 (for the RO unit) + $83,000/year (operating expenses)
Payback period: 3.6 years
Results
– Energy used to concentrate whey reduced by 90 percent ($173,000 annually).
– Steam requirement reduced by more than 95 percent.
– Annual electricity consumption increased 60 MWh (approximately $2,400/year) for the RO equipment, and the annual membrane replacement cost is $64,000.
Project delivered
To concentrate 12 500 L/hr of whey from 6 to 21 percent total dry matter, a conventional triple effect evaporator, not equipped with mechanical or thermal steam recompression and fed by steam produced in a boiler, was replaced with an RO unit. Even though using the membrane filtration unit caused a slight increase in electricity consumption, this technology considerably reduces the need for thermal energy because concentration occurs with the separation of water in its liquid state, which does not require evaporation.
Limitations to the technology
There are limits to the usefulness of membrane filtration in a concentration process. While the details vary from one manufacturer to another, RO is generally used to pre-concentrate whey to up to 25 percent total dry matter. For higher levels of concentration more traditional evaporation methods are required.
4.2 Heat pumps
We are concerned in this guide with closed-loop heat pumps that use an intermediary fluid called the refrigerant. Open-loop systems are found in mechanical vapour recompression technologies (MVR), which are addressed in Section 4.3.
Heat pumps are compression based refrigerating apparatuses designed to transfer heat for heating rather than cooling purposes. They capture thermal energy at relatively low temperatures (cold source), warm it and transfer it to a heat sink.
In the evaporator, the low temperature heat source transfers energy to the refrigerant, which then vaporizes. The compressor temperature and pressure increase while the refrigerant remains vaporized. In the condenser, the refrigerant transfers the accumulated energy to the heat sink. At the condenser outlet, an expansion valve reduces the pressure of the refrigerant. The low-pressure liquid then returns to the evaporator to restart the cycle.
– Applications: Meat, dairy and beverage industries that require heating and cooling. Evaporation and concentration processes.
– Potential: Heat pumps are generally used for refrigeration and air conditioning, but their appeal in the agroprocessing sector is that they can also be used to increase the temperature of a fluid that is several degrees too cool to be usable.
– Limitations: Lack of knowledge and a payback period that usually exceeds 2 years are the main barriers to the industrial use of heat pumps.
Example of an industrial application
Industrial application of the heat pump in one of the sectors covered by this guide is illustrated in the following example.
Heat pump – Meat processing industry
Type of business: poultry processing establishment in Canada
Application: tempering before slicing and cutting frozen quarters
Deployment date: 1987
Cost associated with the heat pump: $165,000 (investment) + $9,500/year (operating costs)
Payback period: 2.9 years
Results
– Annual energy costs reduced by $56,000 (production of hot water by reclaiming heat from the evaporative condenser).
Methodology
In this procedure, a heat pump warms water to a temperature that makes it usable for processes in the establishment by reclaiming and applying heat from condensers that was previously vented outside.
Project delivered
The first floor of the system involves capturing heat from the warm refrigerant (ammonia [NH3] in this case) at the outlets of the refrigeration compressors and preheating the water (from 12°C to 25°C, on average) with heat exchangers that use a water-glycol loop as the intermediary.
The second, and main, floor of recovery uses a heat pump attached to the establishment’s ammonia-based ice production system to heat water that was preheated during the first stage. The heat pump’s refrigerant (R-12) captures the ammonia’s condensation heat and transfers it to the water in the heat pump’s condenser.
The system therefore lets water be heated to between 40°C and 63°C, making it directly usable by the establishment processes.
4.3 Mechanical and thermal vapour recompression
MVR is a technology belonging to the family of “open-loop” heat pump systems that are especially well suited to evaporation processes. MVR allows the latent heat contained in steam, which is often lost in traditional processes, to be recovered. Steam generated by evaporation is recovered by a compressor, which increases pressure and temperature to several degrees above the liquid’s boiling point.
After this steam reaches a high temperature and pressure, it provides a heat source for evaporation as it releases its latent heat. Reclaiming the energy contained in the steam makes significant energy savings possible. In fact, only 30 kWh are required to evaporate 1 m3 of steam, compared to 800 kWh with traditional evaporation.
– Applications: Concentrating milk, brewing beer (wort kettle), concentrating effluents, distillation, separation.
– Potential: In addition to reducing energy consumption, MVR can also significantly cut refrigeration needs (water, cooling tower) and, in some cases, odours.
– Limitations: The principal barrier that this technology must overcome is that it is little known in industrial circles.
– Comment: It is also possible to increase pressure and temperature of the steam produced by evaporation by using a steam ejector. This is thermal vapour recompression (TVR) and, with a smaller investment than for a typical MVR system, it is sometimes possible to reduce the steam requirement by 50 percent.
Example of industrial applications
The following example illustrates industrial applications of vapour recompression (mechanical or thermal) in some of the sectors covered by this guide.
Mechanical vapour recompression (MVR) – Dairy processing industry
Type of business: dairy processing establishment in the United States
Application: concentration of cheese whey
Deployment date: 1988
Payback period: 4 years
Results
– Eliminated the need for steam produced by the site boiler.
– Reduced energy consumption for a net annual savings of $165,000 (annual savings on steam amounting to $211,000 minus $46,000 in annual operating costs for running an additional compressor).
Project delivered
A single effect evaporator that is initially fed by the steam produced in a boiler concentrates the cheese whey. A centrifugal compressor recovers the steam generated by evaporation and brings it, compressed, to a temperature higher than the liquid’s boiling point. Thus compressed, the steam is used as a heat source for the evaporator: The steam sheds its latent heat when it touches the colder liquid, and so provides the heat required for evaporation.
Limitations of the technology
Even though MVR promises considerable energy savings, it usually requires a substantial upfront investment that depends directly on the quantity of water to be evaporated. Consequently, in the case of very dilute liquids, it is advantageous to pre-concentrate the solution prior to evaporation: often, a membrane filtration + MVR evaporation combination proves best.
4.4 Cogeneration – the combined generation of heat and electricity
Traditional systems for generating electricity have an average efficiency of 35 to 40 percent (as high as 55 percent for combined-cycle systems), releasing 60 to 65 percent of the energy content of their fuel into the environment. Cogeneration reclaims this heat loss and applies it to heating or cooling needs. Heating includes the production of steam and hot water. Cooling requires the use of absorption chillers that transform heat into cold. Thus, by simultaneously generating electricity and heat, cogeneration units have a higher overall efficiency that may reach 90 percent. This represents savings on fuel as high as 40 percent compared to electricity and heat production using thermal powerplants and steam boilers.
Figure 4-2 – Generation of heat and electricity with cogeneration
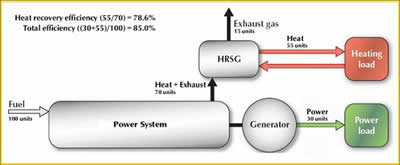
Source: RETScreen® International, clean energy projects analysis – Cogeneration project analysis slide deck
Text Version
Heat recovery efficiency (55/70) = 78.6%
Total efficiency ((30+55)/100) = 85.0%
Fuel (100 units) -> Power System (-> Heat + Exhaust [70 units]
Leads to:
-> HRSG [-> Exhaust gas (15 units)] -> [Heat (55 units)] Heating load -> [back to HRSG])
-> Generator -> (Power [30 units]) Power load
Figure 4-3 – Distribution of industrial cogeneration facilities in Canada
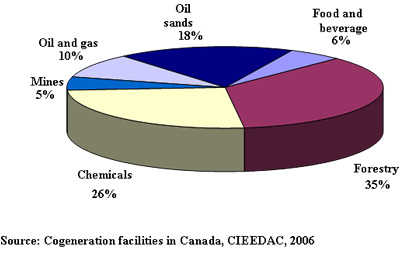
Text Version
Food and Beverages | 6% | |
Forestry | 35% | |
Chemicals | 26% | |
Mines | 5% | |
Oil and Gas | 10% | 4 |
Oil Sands | 18% |
Source: Cogeneration facilities in Canada, CIEEDAC, 2006
Because electricity can be transmitted across great distances more easily than heat, industrial cogeneration facilities are generally located close to the place where the thermal energy will be used. These facilities are also scaled to meet the heat requirements of a specific process. If the quantity of electricity generated is below process requirements, the balance must be purchased from the local grid. Conversely, if a surplus of electricity is generated, it can be sold to the grid. However, this presupposes that the grid hook-up complies with very strict standards and that rules exist for buying and selling electricity. With the recent deregulation of the electricity market, completed in some provinces and ongoing in others, industry can henceforth envisage building cogeneration plants and having the option of selling any excess electricity to the grid.
In Canada, existing cogeneration facilities are found in the forestry products sector (which operates many steam turbines), in chemicals, and in the oil sands (where the most powerful facilities are installed). Cogeneration systems are also found at 15 establishments in the food and beverage sector (corn processing, distilleries, breweries, sugar refineries, poultry processing, etc.).
In 2005, the output from the cogeneration facilities that provide heat at establishments in the food and beverage sector was 351 megawatts electric (MWe). Their average efficiency was 80 percent and their average heat to electrical power ratio (HTPR) was 6.3. This means that, for each kilowatt-hour of electricity generated, 6.3 kWh of usable heat was produced by these facilities.
Main components and characteristics of a cogeneration system
A cogeneration facility consists of the following four main components:
- the prime mover, generally a turbine or a combustion engine
- an electric generator powered by the prime mover
- a new heat recovery boiler to produce steam from the energy contained in the exhaust gas of the turbine or combustion engine. Energy recovery can be maximized by installing a standard economizer at the outlet of the heat recovery boiler (the temperature of the combustion gas, which ranges from 120°C to 150ºC, depending on the fuel, can also be reduced). If the process requires a significant volume of hot water, a condensing economizer can follow, or replace, the economizer (the temperature of the combustion gas can then be lowered to 50°C or 60ºC).Footnote 15
- a control system
The most commonly used energy sources are steam (steam turbine) and natural gas (gas-fired engine and turbine), though some applications use diesel and biogas.
If the HTPR (heat to power ratio) varies throughout the day or across seasons, any change in the quantity of electricity generated, or any purchase of electricity, can result in a significant loss of earnings. Therefore, it is preferable to adjust the HTPR to the needs of the site by using an additional burner at the intake of the heat recovery boiler or a supplementary boiler.
Optimizing a cogeneration system (i.e. adapting it to heat requirements) yields the following principal benefits:
- Economic and environmental benefits:
- increase in the overall efficiency with which fuel is converted into heat and electricity
- access to income from the sale of excess electricity to the grid
- reduced cost of treating effluents and disposing of waste when biogasFootnote 16 is used, increasing the profitability of the system
- reduced atmospheric emissions, especially carbon dioxide (CO2) and nitrogen oxides
- Greater security of electricity supply: cogeneration reduces the risk of production disruption in the event of a blackout.
- Decentralized electricity generation near the point of use limits losses on transmission lines.
- The application has been tested in most industrial sectors around the world, especially in several processes in the food and beverage industry as well as agriculture.
In general, cogeneration requires a large investment with a payback period of four to five years. The cost of purchasing the equipment and connecting it to the process and the electricity grid must be added to the cost of building a chamber or structure to attenuate the noise produced by gas turbines and engines. Therefore, any decision to build a cogeneration plant should account for the following elements:
- the annual thermal energy and electricity needs of the process, their seasonal variations and forecasts of future developments
- the potential for energy savings – A detailed energy audit designed to optimize energy use at the establishment should be performed before launching any cogeneration project. It may, in fact, happen that, after cogeneration is in place, it becomes more difficult to further improve energy efficiency.
- the type of fuel used and forecasts of trends in its price and the price of electricity
- the cost of investing in equipment and the civil infrastructure
- existing incentive programs
Tax savings under Class 43.1 and Class 43.2 of the Income Tax Regulations
Cogeneration systems that generate electrical energy and heat that is exported from the system for useful purposes are eligible for tax savings under Class 43.1 or Class 43.2 of the Income Tax Regulations. These tax measures allow accelerated deduction of capital costs at:
– Thirty percent per year on a declining basis if the heat rate does not exceed 6000 BTu/kWh (6330 kilojoules [kJ]/kWh) in the case of Class 43.1 or.
– Fifty percent per year on a declining basis if the heat rate does not exceed 4750 Btu/kWh (5011 kJ/kWh) and the equpment is acquired after February 22, 2005 and before 2020 in the case of Class 43.2.
For more information about tax savings for clean energy generation and energy conservation equipment, consult the Class 43.1 Technical Guide and Technical Guide to Canadian Renewable and Conservation Expenses (CRCE), or contact the Class 43.1 and 43.2 Secretariat.
*For the purposes of Class 43.1 and 43.2, heat rate is defined as F/(E+H/3413), where F is the higher heating value (HHV) of the eligible fossil fuel consumed in a year, E is the gross electrical energy generated in a year and H is the net heat exported from the system for useful purposes in a year.
Evaluation of cogeneration projects
The RETScreen® software cogeneration model makes it possible to assess energy generation, life-cycle cost, emissions cuts, financial viability and risks associated with electricity, heat and cold generation projects in one or many buildings and in industrial processes. The model makes possible feasibility studies that account for a vast array of renewable and non-renewable fuels and contains a database of climate and product data (e.g. reciprocating engines, gas turbines, combined-cycle gas turbines, steam turbines, fuel cells, microturbines, boilers, compressors, absorption cycle heat pumps, etc.).
This cogeneration model can be downloaded free of charge from the RETScreen® International Web site: www.retscreen.net.
RETScreen® International is administered by Natural Resources Canada’s CanmetENERGY Technology Centre in Varennes.
Technology | Fuel | Typical size (MWe) | Electrical efficiency | Heat to power ratio (HTPR) |
Overall efficiency |
---|---|---|---|---|---|
Spark ignition reciprocating engines | Natural gas Biogas Diesel |
0.003 to 6 | 25 to 43 % | 1:1 to 3:1 | 70 to 92 % |
Compression ignition reciprocating engines | Natural gas Biogas Diesel Heavy fuel oil |
0.2 to 20 | 35 to 45 % | 0.5:1 to 3:1* | 65 to 90 % |
Combined-cycle turbine | Natural gas Biogas Diesel Heavy fuel oil |
3 to 300 | 35 to 55 % | 1.1:1 to 3:1* | 73 to 90 % |
Open-cycle turbine | Natural gas Biogas Diesel |
0.25 to 50+ | 25 to 42 % | 1.5:1 to 5:1* | 65 to 87 % |
Back-pressure steam turbine | None | 0.5 to 500 | 7 to 20 % | 3:1 to 10:1+ | Up to 80 % |
Extraction steam turbine | None | 1 to 100 | 10 to 20 % | 3:1 to 8:1+ | Up to 80 % |
Source: COGEN Europe (European Association for the Promotion of Cogeneration)
*For these systems, higher heat to power ratios can be obtained by adding an additional burner at the outlet of the engine or turbine.
Example of an industrial application
An industrial application of cogeneration in one of the sectors covered by this guide is illustrated in the following example.
Cogeneration, or combined generation of electricity and heat – Meat processing industry
Type of business: a poultry slaughterhouse and processing establishment in Canada (capacity 300 000 chickens per day)
Application: simultaneous generation of electricity, steam and hot water using natural gas
Deployment date: 1999
Cost of investment: approximately $6 million
Payback period: 5.5 years
Results
Installation of a 5-MWe natural gas turbine made the following measures possible:
– reduce electricity cost from $0.065/kWh to $0.05/kWh (more than 20 percent)
– increase the security of the establishment electricity supply by generating a substantial share of the electricity used
– reduce natural gas consumption approximately 4 percent to achieve an overall efficiency rate (generation of electricity and heat) of 86 percent
Methodology
The slaughter and processing of poultry imposes strict sanitary conditions. Large volumes of hot water and steam are used in the processes and to clean equipment. Prior to the cogeneration project, hot water was produced by several pieces of equipment, such as an interconnected network of boilers and heat pumps. A great deal of electricity was also required to refrigerate the packaging rooms and for freezing. Every day, the establishment consumes 2270 m3 (500 000 imperial gallons, or 1 892 706 L) of hot water and, in the summer, up to 9.5 MW of electricity. With cogeneration, it is possible to rationalize the production of thermal energy while producing electricity to power the establishment’s refrigeration system.
Project delivered
The approach implemented generates electricity, steam and process hot water with a cogeneration plant. The delivered system features the elements listed below:
– 5.2-MWe natural gas turbine
– at the outlet of the gas turbine, an additional burner and a heat reclamation system for producing steam for the establishment (29 484 kg/h, 125 pound-force per square inch gauge)
– at the outlet of the steam generator, a direct contact economizer capable of heating 1360 L (300 imperial gallons) of water to 49ºC (120ºF) every minute
– a separate building for the cogeneration unit, thereby avoiding significant renovation and soundproofing costs in the establishment
4.5 Anaerobic treatment of effluents and wastes
The anaerobic process is one of the most promising avenues for treating industrial effluents and waste with a substantial load of organic matter. In the absence of air and oxygen, some bacteria transform organic residues from vegetable, animal and chemical sources into biogas (consisting of methane and CO2), which can be used as fuel to replace natural gas and fuel oil. Depending on the specifics, the process is called anaerobic treatment, digestion or fermentation. These designations are equivalent and we have opted for the term anaerobic treatment (AT) in this guide.
A wide variety of organic compounds can be treated anaerobically: carbohydrates (starch, sugar, cellulosic materials), fats and oils, and proteins. AT is well known in Europe and Asia, where it is estimated that hundreds of such industrial systems are in operation, but in North America the process is still poorly represented, with a mere 12 percent of the world’s facilities.
Figure 4-4 – Breakdown of industrial anaerobic treatment facilities in Europe
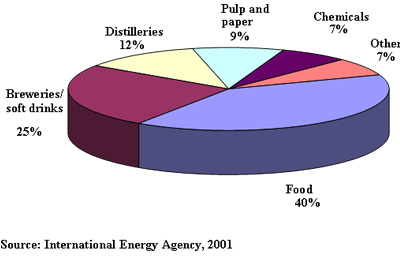
Source: International Energy Agency, 2001
Text Version
Food | 40% |
Breweries / Soft Drinks | 25% |
Distilleries | 12% |
Pulp and Paper | 9% |
Chemicals | 7% |
Other | 7% |
Source: International Energy Agency, 2001
In Europe, approximately 75 percent of industrial AT applications are in food and beverage industries, 9 percent in pulp and paper, and 7 percent in chemicals. In Canada, existing facilities are mostly applications that reclaim manure in the agricultural sector. AT is also used at several establishments in the food and beverage industry and is finding wider application in the reclamation of residues at pulp and paper mills.
Anaerobic treatment – principles and characteristics
In the food and beverage sector, this technology was developed to pretreat water that has a high organic content. The process converts approximately 90 percent of the organic load into biogas while producing fertilizer as a by-product. The main steps in the process are as follows:
- a physical (shredding), chemical (hydrolysis) or thermal (pasteurization) pretreatment is sometimes required prior to anaerobic treatment
- organic matter (dissolved or suspended in water) is fed into a reactor,Footnote 17 where, in the absence of oxygen, anaerobic bacteria convert it into biogas and residues (solid or liquid) that can be used as fertilizer
- separation of the products (biogas and solid or liquid residues) can occur in the reactor itself or in a separate piece of equipment downstream
- raw biogas, which contains between 50 and 80 percent methane (the main element of natural gas) and between 20 and 50 percent CO2, presents a considerable calorific potential
Biogas also contains trace amounts of hydrogen sulphide (H2S). If it is too prevalent, the H2S must sometimes be removed from the biogas before the biogas is used as a fuel.
The solid residue can be used as wet fertilizer, can be dehydrated and used as dry fertilizer, or can be composted, buried or incinerated.
In certain cases, an organic residue remains after AT. This residue can be treated by an ordinary AT. The final wastewater can then be discharged into the environment or the municipal sewage system at a cost that is considerably below what it would have been without AT.
Food | Fluid milk Dairy products (cheese, butter, cream, yogurt, ice cream, whey) Products of slaughterhouses and meat processing Vegetables (canned or frozen) Fish, seafood, and by-products Products of corn, grain, potatoes, and oilseeds (oils, starch, margarine) |
---|---|
Beverages | Beer Soft drinks Distillery spirits Fruit juices and products Wine |
The principal benefits of AT are demonstrated in the following examples.
- Economic and environmental benefits:
- a reduction in the effluent discharged into the environment or into the municipal sewage system and in the odours given off by organic waste
- the generation of biogas, an energy source that can be used as a fuelFootnote 18 in boilers or in the establishment’s cogeneration system as a replacement for fossil fuels (natural gas or fuel oil)
- The production of solid residues that can be used as fertilizer.
- An application that has proven its worth around the world in several food and beverage industry processes, such as breweries, distilleries, dairies and slaughterhouses.
4.6 New modes of heat transfer
Traditional modes of heating and cooking food in hot air ovens or by contact with heated surfaces are now complemented by new high-efficiency modes based on electrotechnologies. These techniques include infrared, high frequency and microwave radiation, as well as ohmic and induction heating.
The principles behind these different modes of heat transfer vary considerably from one to another, but they are all designed to heat the product rapidly and efficiently, while meeting taste and nutritional criteria.
The principal benefits of these technologies are demonstrated by the following examples:
- high energy yields (up to 95 percent)
- direct heating with no intervening fluid
- quick response time for start-up, stop and adjustment
- precise temperature adjustments
- oil-free cooking processes
- minimal loss of product mass
4.6.1 Infrared radiation heating
Infrared radiation (IR) heating technology uses electric resistors and/or ceramic natural gas elements, which are heated to the required temperature (several hundred degrees Celsius) for them to give off the desired radiation type, be it short-, medium- or long-wave IR. The primary characteristic of IR is that it is usually absorbed at the surface of the product, causing a rapid rise in temperature.
– Applications: Cooking and roasting meat. This technology provides an interesting alternative to traditional methods that use hot air ovens or oil-based rotisseries.
– Limitations: IR is ideal for treating surfaces and heating products arranged in thin layers. It cannot heat thick products uniformly, and may even cause thermal degradation.
Example of an industrial application
Industrial applications of IR radiation treatment in several of the sectors covered by this guide are illustrated in the following example.
Infrared radiation (IR) treatment – Meat processing industry
Type of business: poultry processing establishment in Germany and a meat processing establishment in the Netherlands
Application: cooking chicken fillets and pork spare ribs
Deployment date: 1998
Economic data: not available
Results
– Cooking chicken fillets: The energy bill was reduced 78 percent (annual benefit of $68,200) while production capacity doubled (500 kg/hr instead of 250 kg/hr).
– Cooking pork spare ribs: The energy bill was reduced 67 percent (annual benefit of $137,400) while production capacity increased 35 percent (950 kg/hr up from 700 kg/hr).
Technological benefits: With IR, energy is transmitted directly to the product, thus precluding the need for an intermediary fluid as in an ordinary broiler. The operation is faster and easier to control. Also, cooking with IR does not require oil, which needs to be replaced regularly in traditional processes, thus reducing costs even more.
4.6.2 Microwave and high-frequency radiation
These electrotechnologies make it possible to heat, directly and rapidly, without an intermediary, such poorly conductive substances as agroprocessing products. While they yield very different results in practice, both technologies are based on the same principle: an alternating electrical field stimulates movement of molecules (especially water and fats), which causes heat. The technologies exist as continuous applications in the form of tunnels and as discontinuous (or batch) applications in the form of closed chambers, and can be procured from several equipment vendors.
The treatment characteristics, including heating uniformity, depend on the nature, shape and thickness of the product. Preliminary tests at a pilot establishment are required to determine optimal operating conditions. Among current technological solutions, we find that intermittent microwave (MW) or high frequency application prevents product overheating, and moving the product in the chamber promotes uniformity of treatment.
– Applications: tempering and cooking food, bacterial control in frozen products (meats, fish), pasteurization of packaged goods (prepared foods).
Examples of industrial applications
Industrial applications of MW radiation treatment in several of the sectors covered by this guide are illustrated in the following examples.
Microwave radiation (MW) treatment – Meat processing industry (first example)
Type of business: turkey processing establishment in the United States
Application: tempering frozen turkeys prior to processing
Deployment date: not available
Economic data: not available
Results
Traditional methods of tempering the flesh reduce mass: With hot air, the reduction is approximately 1 to 3 percent and with hot water-based processes, it is as much as 5 percent. This weight loss is negligible when the flesh is tempered by using MW radiation treatment.
Technological benefits
During MW radiation treatment, all the energy is absorbed by the meat. There is no energy lost by having to heat an intermediate fluid, as in traditional hot air and oil-based methods, and treatment duration is considerably reduced.
Microwave radiation (MW) treatment – Meat processing industry
Type of business: meat processing establishment in the United States
Application: tempering frozen quarters prior to slicing and cutting them
Deployment date: not available
Economic data: not available
Results
– Using traditional methods (tempering room), it took 2 to 5 days to raise the temperature to -2°C. With MW technology, the time was reduced to a few minutes, adding flexibility to production management.
– Loss of product during slicing and cutting operations was reduced 20 percent because of better temperature control and more uniform product temperature.
Technological benefits
Same as previous example.
4.6.3 Ohmic heating
Ohmic heating, also known as Joule and resistive heating, consists of passing an electric current directly through the item to be heated. It can be applied to liquids (provided they are reasonably conductive) that are generally difficult to treat (heat-sensitive, very viscous, messy, etc.) and allows rapid heating of large volumes with a great deal of control.
Recent success in the development of ohmic treatment of liquids has led to the marketing of the first generation of equipment and triggered work on ohmic cooking of meat products.
– Applications: Heating and sterilizing milk, fruit juice, beer and meat sauces.
– Limitations: Currently, the technology appears very difficult to apply to solid matter, such as pieces of meat. However, very promising results were recently obtained in tests on ham emulsions: an increase in the quality of the product while reducing cooking time by as much as 75 percent.
4.6.4 Induction heating
Heating by electromagnetic induction involves placing the product in a fluctuating magnetic field. This creates Foucault (eddy) currents in the material, which induce Joule heating. From a technical perspective, heat may be applied directly to the product, which heats it from inside, or indirectly to a surrounding cover made of metal or other material, heating it by induction. Low system inertia allows precise control of the temperature.
– Applications: Heating and sterilizing liquids (milk, fruit juices), doughs and pastes.
Example of an industrial application
An industrial application of induction heating in one of the sectors covered by this guide is illustrated in the following example.
Induction heating – Dairy processing industry
Type of business: dairy establishment in Canada
Application: high temperature pasteurization (ultra high temperature [UHT] process)
Deployment date: 1996
Cost of investment: $855,000 (UHT pasteurizer)
Payback period: 3.3 years
Results
Reduction in energy consumption resulting in net annual savings of $259,000.
Technological benefits
Compared to traditional methods of pasteurization that use thermal energy from a steam boiler, the induction process is 17 percent more efficient.
4.7 Cold process pasteurization and bacterial control
Pasteurization of a food product is the process of eliminating or deactivating microorganisms that may affect quality. Depending on the product and the technique used, the classic process consists of heating the product to a temperature of between 60°C for beer and 72°C for milk, or even higher, before or after the product is conditioned in a plate cooler or a tunnel pasteuriser. However, hot process pasteurization has the disadvantage of being a major consumer of energy and it may affect the organoleptic properties (principally taste) and nutritional value of the product.
To avoid these problems, new techniques in cold process treatment developed in recent years all share the feature of rapidly reducing the microbial community at a moderate temperature. These techniques have a wide range of applications in the agroprocessing industry, from product pasteurization to disinfection. Over time, their deployment is expected to gain wide acceptance in Canada.
More advanced techniques, such as high pressure, ultraviolent, microfiltration and ultrafiltration, are already being used and, in the next few years, the use of other technologies, such as electron beams and magnetic and electrical fields, will increase.
The principal benefits of cold-process pasteurization techniques are as follows:
- reduced consumption of water and energy
- significant extension of the shelf life of treated products
- no product degradation caused by heat (the taste remains very close, or even identical, to that of the untreated product, preservation and stabilization of vitamin content, etc.)
4.7.1 Microfiltration and ultrafiltration treatment
Microfiltration and ultrafiltration are membrane filtration methods that make it possible to selectively separate bacteria and other materials. Marketed in Canada, they have already been used in the dairy and beverage (beer and fruit juices) industries for both pasteurizing and clarifying liquids. By coordinating membrane pore size with the product being processed, these two operations may even be performed simultaneously, resulting in considerable energy savings because one of the steps in the process is eliminated.
– Applications: Pasteurizing products and controlling water-borne bacteria
4.7.2 High-pressure (hyperbaric) treatment
High-pressure, or hyperbaric, treatment consists of exposing the product, whether packaged or not, to high hydrostatic pressure to eliminate pathogens and microorganisms. At present, this technology can be applied to liquids (fruit juices) and some solids (purées, jellies, deli meats). It has found widespread acceptance only in Japan.
– Applications: In the fruit processing sector, this method preserves all the qualities of fresh fruits for about one month.
It shows some potential for fluid milk and cheese processing. However, work continues on controlling its impact on the natural enzymes in milk and the texture of the final product.
– Potential: The use of high pressure allows the development of products with interesting characteristics in texture, appearance and taste. It also improves the performance of some processes, for example, by driving solutes into products, and freezing and thawing products with a minimum of sweating.
Example of an industrial application
An industrial application of hyperbaric treatment in one of the sectors covered by this guide is illustrated in the following example.
High-pressure (hyperbaric) treatment – Meat processing industry
Type of business: meat processing establishment in Spain (21 hr/day)
Application: pasteurization of cooked and vacuum-packaged sliced ham (625 kg/hr)
Deployment date: 1998 (new production line)
Cost of investment: $1.4 million for the hyperbaric chamber
Results
The annual energy bill was reduced by approximately $10,500 (annual electricity consumption of 26 kW – 6300 hr at $0.064/kWh).
Technological benefits
The client based the choice on three criteria: maintaining the organoleptic integrity of the product; ensuring a shelf life of at least one month; low operating cost.
4.7.3 Ultraviolet treatment
In the United States, the Food and Drug Administration (FDA) recently approved ultraviolet (UV) treatment as an alternative to the heat process pasteurization of juices. This treatment is of great interest to apple juice producers, who do not have the infrastructure to apply heat treatment to small volumes of output. The technology is also approved for other juices, whether fresh or from concentrate.
– Applications: Pasteurization of juices and whey (high-density UV) and control of water-borne bacteria
– Limitations: UV technology can be used on liquids that are relatively clear. It cannot be used on milk because the taste may be affected. UV treatment of whey necessitates high intensities of UV power, while low intensities are adequate for disinfecting water.
4.7.4 Treatment with electric or magnetic fields
Recent advances in the area of electric and magnetic fields (generally pulses) have shown that these techniques can deactivate microorganisms and microbiota, suggesting a real potential for the agroprocessing industry.
– Applications: Pasteurization of many products, both liquid and solid (meat products, cheeses, cakes, fruits and vegetables, egg based products, purées, sauces, milk, juices, syrups), treating products in opaque packaging.
– Limitations: While they are progressing rapidly and showing great potential, these processes are in their early development days (development and fine-tuning phase).
4.7.5 Electron beam treatment
In the United States, the FDA recently approved the use of gamma rays (from a cobalt-60 or cesium-137 source), X-rays below five megaelectron volts (MeV), and electron beams below 10 MeV.
Electron beam technology, in use for over 40 years for sterilizing medical equipment. It has made significant strides in recent years, with developments in its potential for sterilizing and pasteurizing a wide variety of products in the agroprocessing industry. It is also considered to be the most promising of the pasteurization technologies based on ionizing radiation.
– Applications: Processing meats, dairy products and packaged food products.
– Limitations: The main hurdle this technology must overcome is public perception of irradiated food products.
4.8 High-efficiency homogenization valves
Homogenization consists of breaking globules suspended in a liquid into smaller particulates to create a mixture that is more homogeneous and stable. The operation takes place in a homogenizer in which the liquid is forced through openings or valves under pressure.
In the dairy industry, the purpose of homogenization is to break globules of milk fat into smaller particles to distribute them evenly throughout the milk. This process stabilizes the product and, in particular, keeps the fatty matter from rising to the surface as cream. It also confers physical and organoleptic properties that make the product attractive on the fluid and industrial milk markets.
In recent years, manufacturers have developed new generations of high-efficiency valves that work at lower pressures, reducing equipment electricity consumption 15 to 30 percent while maintaining the same quality of homogenization.
– Application: Homogenizing milk.
– Potential: The use of high-efficiency homogenization valves makes it possible to either reduce energy consumption by reducing pressure to 1100 pounds per square inch, for example, or to increase homogenization quality by continuing to operate at the traditional higher pressure of 1350 psi, thereby increasing the shelf life of the homogenized milk.
Example of an industrial application
An industrial application of high-efficiency homogenization valves in one of the sectors covered by this guide is illustrated in the following example.
High-efficiency homogenization valves – Dairy processing industry
Type of business: dairy establishment in Canada (12 hr per day)
Application: homogenization of 20 000 L/hr of 3.25 percent milk
Deployment date: 2001
Cost of investment: $12,900
Payback period: 2.5 years
Results
For the same quality of homogenization, reducing the operating pressure (from 170 bars to 114 bars) and the electrical power (from 111 kW to 75 kW) resulted in an annual decrease in electricity consumption of 132 500 MWh ($5,300).
Methodology
The project consists of replacing the original valves with high-efficiency valves on an existing machine. This is probably the most common situation because homogenization equipment has a very long lifespan.
Technological benefits
High-efficiency valves work at lower pressures, reducing equipment electricity consumption. In addition to directly reducing electricity consumption, the use of more efficient valves also contributes to limiting the site’s peak power demand.
Page details
- Date modified: