The First Canadian Smart Remote Microgrid: Hartley Bay, BC
Download full document (PDF, 2 MB)
Introduction
There are 292 remote communities in Canada, many of which rely on diesel for electricity generation and subsequently have high costs of electricity (up to 10 times compared to the main electric grid), mostly due to the cost of fuel transportation and delivery. Moreover, fuel costs are also volatile, difficult to forecast, and likely to increase. These high costs drain financial resources which could otherwise be allocated to other priority areas. Since many remote communities are growing rapidly and building construction is expanding, new electrical infrastructure is becoming necessary. This provides opportunities to include or increase renewable energy supply in communities, and to ensure that new buildings are designed to reduce demand and facilitate the integration of renewables on the grid. However, it is difficult to convince these remote communities to integrate high penetrations of intermittent renewable generation because of its past inability to economically offset diesel consumption. Recently, with the revolution of smart grid technology (smart controls and advanced metering and monitoring), it is possible to quantify the full potential of renewables and bring real cost savings to these communities. In communities like Hartley Bay, a smart grid with demand response will play a crucial role in maximizing the use of renewables.
Integrating Renewable Resources: Old vs New Approaches
During the last 10 years, CanmetENERGY and national and regional partners have been working on improving the performance of remote Microgrids and reducing their dependence on diesel fuel for electricity generation. The old approach used in integrating renewable resources in remote communities included conducting energy audits of the community prior to sizing and integrating renewable resources (most of the time under-sized); monitoring the system performance (mainly renewables and diesel gensets) followed by assessing renewable and diesel performance; and finally, investigating and integrating suitable energy conservation measures. Such an approach usually led to limited system performance improvement.
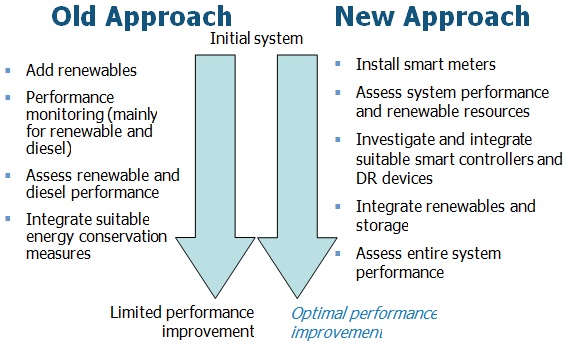
Figure 1: Old Approach vs. New Approach
Text version
Integrating Renewable Resources: Old vs New Approaches
The old approach for integrating renewable resources in remote communities was consisting of adding renewables; performance monitoring (mainly for renewable and diesel); assessing renewable and diesel performance; and integrating suitable energy conservation measures. This approach was conducting to limited performance improvement. The new approach for integrating renewable resources is to install smart meters; assess system performance and renewable resources; investigate and integrate suitable smart controllers and demand response devices; integrate renewables and storage; and assess entire system performance. This new approach leads to optimal performance improvement.
Gaps in the old approach have been identified as the following:
- Inefficient/absence of energy management systems in remote grids.
- Improper balancing of supply and demand affecting quality of power.
- Less than expected reduction in fuel consumption after renewable resources integration due to the improper sizing of renewables or absence of storage and energy management capabilities.
It is expected that accurate system performance assessment for remote grids using real-time collected data would result in a number benefits:
- Implementation of efficient energy conservation measures for proper management of load and diesel generator operation that will lead to major improvements (reductions) in fuel consumption.
- Development of efficient diesel dispatch and load control strategies.
- Optimal selection and sizing of renewable and storage technologies which will facilitate the integration of high penetration of renewable resources into remote grids.
A new approach has been proposed for remote microgrids system performance enhancement and renewable resources integration that includes installing smart meters followed by system monitoring and real-time data collection (preferably for at least 1 year); next, assessing system performance and renewable resources followed by investigating and integrating suitable smart controllers and DR devices; then, selecting and integrating the appropriate technology and size for renewables and storage (if required); and finally conducting entire system performance assessments.
Case Study: The Smart Remote Community of Hartley Bay
About Hartley Bay
The Village of Hartley Bay, located approximately 650 km North West of Vancouver, BC, is a remote, off-grid coastal community in the Gitga’at Nation. The community is home to 170 residents living in 82 buildings: 62 residential and 20 commercial/mixed-use. There are three generators supplying the electricity to Hartley Bay: two 420 kW and one 210 kW. The system consists of a 600 V bus at the generators which is stepped up to 25 kV for distribution (approximately 2 km of lines) and stepped down to 120/240/208 V for residential and commercial single and three phase loads with 25 kVA and 50 KVA transformers. The community has historically used up to 2 GWh of electric energy annually at a levelized cost of approx. $0.67 per kWh. The current generation setup had cost the band upwards of $500,000 per year. The community is now operating a smart microgrid system and is interested in finding additional, innovative ways to improve the efficiency of the generation system that will in turn reduce the community’s electrical demand, energy consumption, greenhouse gas (GHG) emissions, and costs.
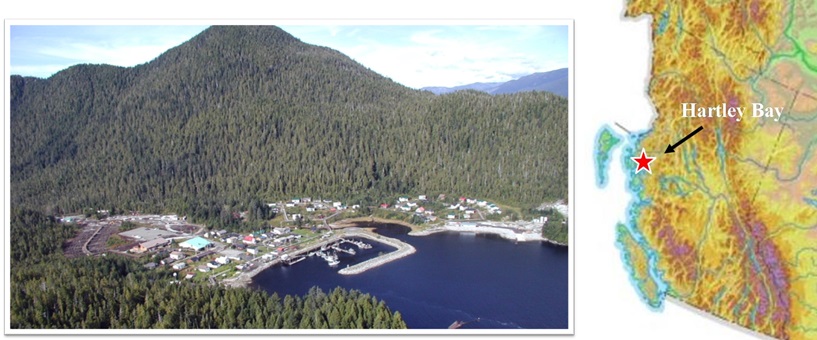
Figure 2: Hartley Bay Community, the home community of the Gitga’at First Nation people, British Columbia (Credit: Pulse Energy, Left) and Location of the Village of Hartley Bay (Right)
Text version
About Hartley Bay
On the left side, it is an aerial picture of the Hartley Bay Community, the home community of the Gitga’at First Nation people, in British Columbia. We can see mountains in the background and a village near a river. On the right side, a star identifies the location of the Village of Hartley Bay on the map of Western Canada.
Remote Grids System Performance Monitoring and Assessment
Since 2008, Hartley Bay has been engaged in an energy management initiative aimed at reducing GHG emissions. One focus of this initiative is managing all aspects of the electrical network including generation, distribution, and demand. Several energy management initiatives have been implemented including installation of a wireless network of smart meters; monitoring of energy use in real-time using an energy management information system (EMIS); lighting, heating and HVAC retrofits; and hiring of local energy coordinators to manage projects and engage the community.
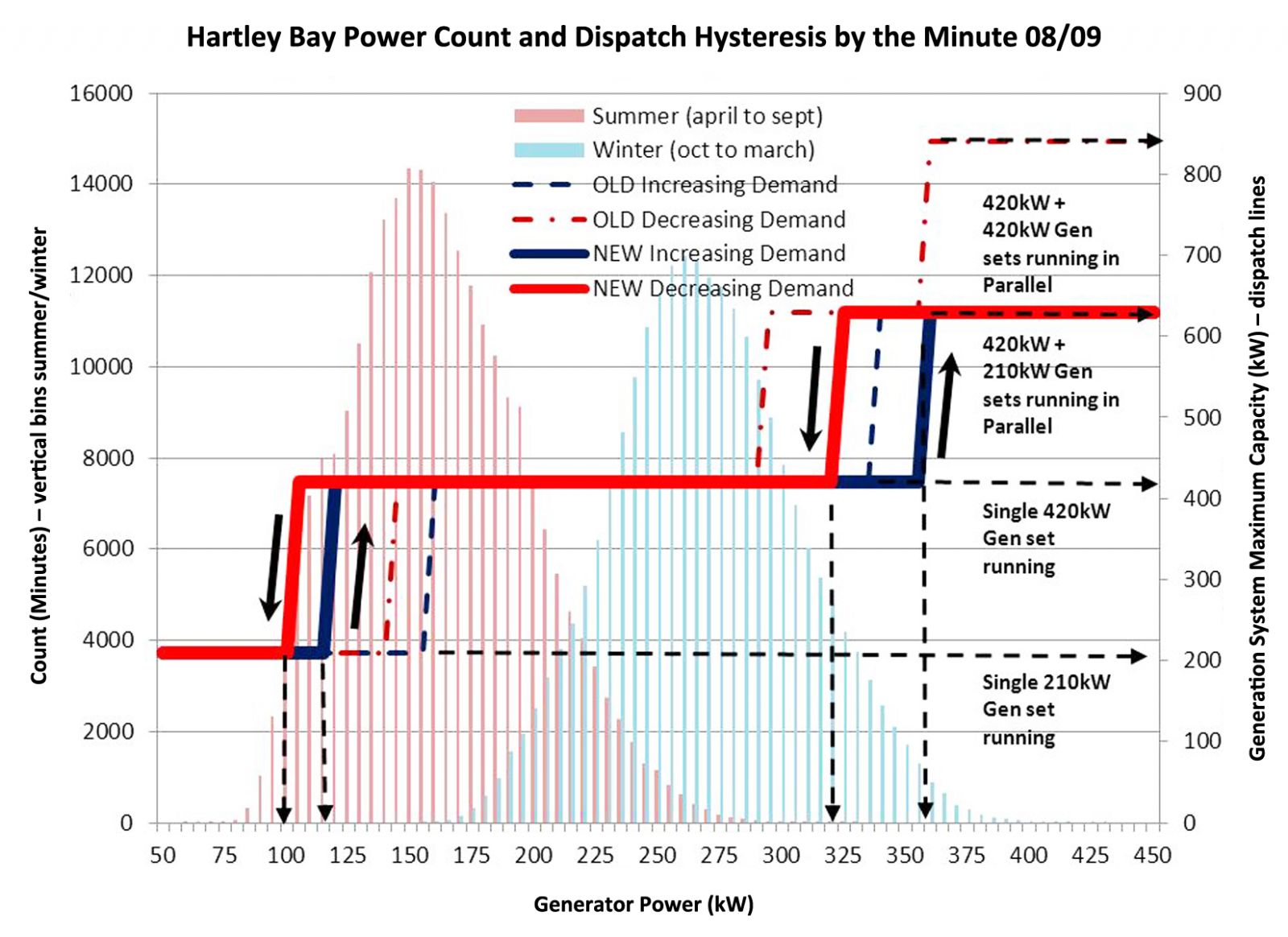
Figure 3: Optimizing the diesel dispatch for the Hartley Bay genset (Credit: Pulse Energy)
Text version
Remote Grids System Performance Monitoring and Assessment
This image is a graph representing the Hartley Bay power count and dispatch hysteresis by the minute. The left axis is in thousands of minutes of operation and is correlated to the two vertical distribution bars charts. The red bars represent the electrical demand occurrence from April to September while the blue bars represent the electrical demand occurrence from October to March. The right hand axis is correlated to the generator dispatch lines in kW. The dotted lines are the original dispatching for the generators while the solid lines represent the new dispatching lines programmed into the generator controller. The red lines represent the falling demand dispatching; in other words, the generator dispatching when the demand decreases from higher to lower demand levels. The blue lines represent the rising demand dispatching; in other words, the generator dispatching when the demand increases from lower to higher demand levels. Since the 210 kW generator was found to be the least efficient generator, the main target was to reduce the periods of operation of this generator. Therefore, the following changes have been applied to the dispatch settings: dispatching for the 210 kW to 420 kW generators was moved down to 120 kW from 160 kW for the 420 kW generator pickup and down from 145 kW to 105 kW for the 420 kW generator dropout.
In October 2009, precision fuel flow sensors were installed on the three generators and generator efficiency, defined as litres of fuel consumed per kWh of electricity produced, was determined. The 420 kW generators were found to have the highest efficiencies (0.27 L/kWh or 34 per cent) while the 210 kW generator had the lowest efficiency (0.49 L/kWh or an average of 19 per cent). It was concluded that, in order to maximize the efficiency of the generation system and minimize fuel consumption, the 210 kW generator should be run as little as possible. One way to achieve this is by modifying the diesel dispatch setting as shown in Figure 3.
Demand Response (DR) System Installation
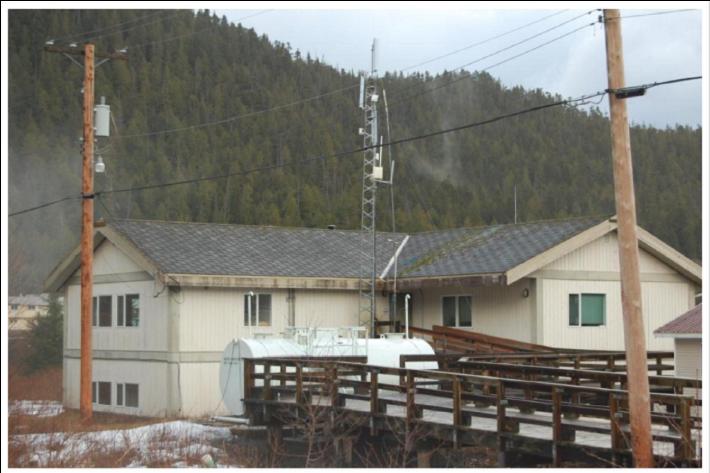
Figure 4: Paging antenna tower (Credit: Pulse Energy).
Text version
Demand Response (DR) System Installation
We can see on this picture a paging antenna tower next to a commercial building in the Village of Hartley Bay.
A DR system was proposed to shed community loads during peak periods in order to keep demand below 360 kW and therefore avoid dispatching of the 210 kW generator. In the spring of 2010, a DR system consisting of twenty variable thermostats and twelve 30-amp load controllers was installed in several commercial buildings in the community. An audit of the facilities determined that the loads with the highest energy usage and the lowest chance of occupant disturbance were baseboard heaters, hot water heaters, and HVAC systems. In order to further minimize the chance of occupant disturbance, a manual DR override was also installed. The current DR setup in Hartley Bay allows for both manual and automatic decision-making. The DR system is capable of shedding up to 15% (61.3 kW) of the maximum demand by adjusting wireless variable thermostats and load controllers on hot water heaters and ventilation systems in commercial buildings. The system was found to be successful in reducing demand by up to 35 kW during the DR event period.
Benefits and Lessons Learned
- Reconfiguring the diesel dispatch set points resulted in an estimated savings of 77,000 litres of diesel per year ($77,000/year)
- It is expected that, in the community of Hartley Bay, unlocking the full potential of future DR programs could produce additional annual fuel savings of up to 27,000 L, or 5% of total consumption.
- Abnormal operational issues can be more efficiently identified using real-time measurements than using conventional system audits.
- The presence of a local champion at the community who can communicate the importance of the project to the community is essential for the success of the project.
- By targeting building with the highest energy consumptions, there is a greater chance for efficiency improvement.
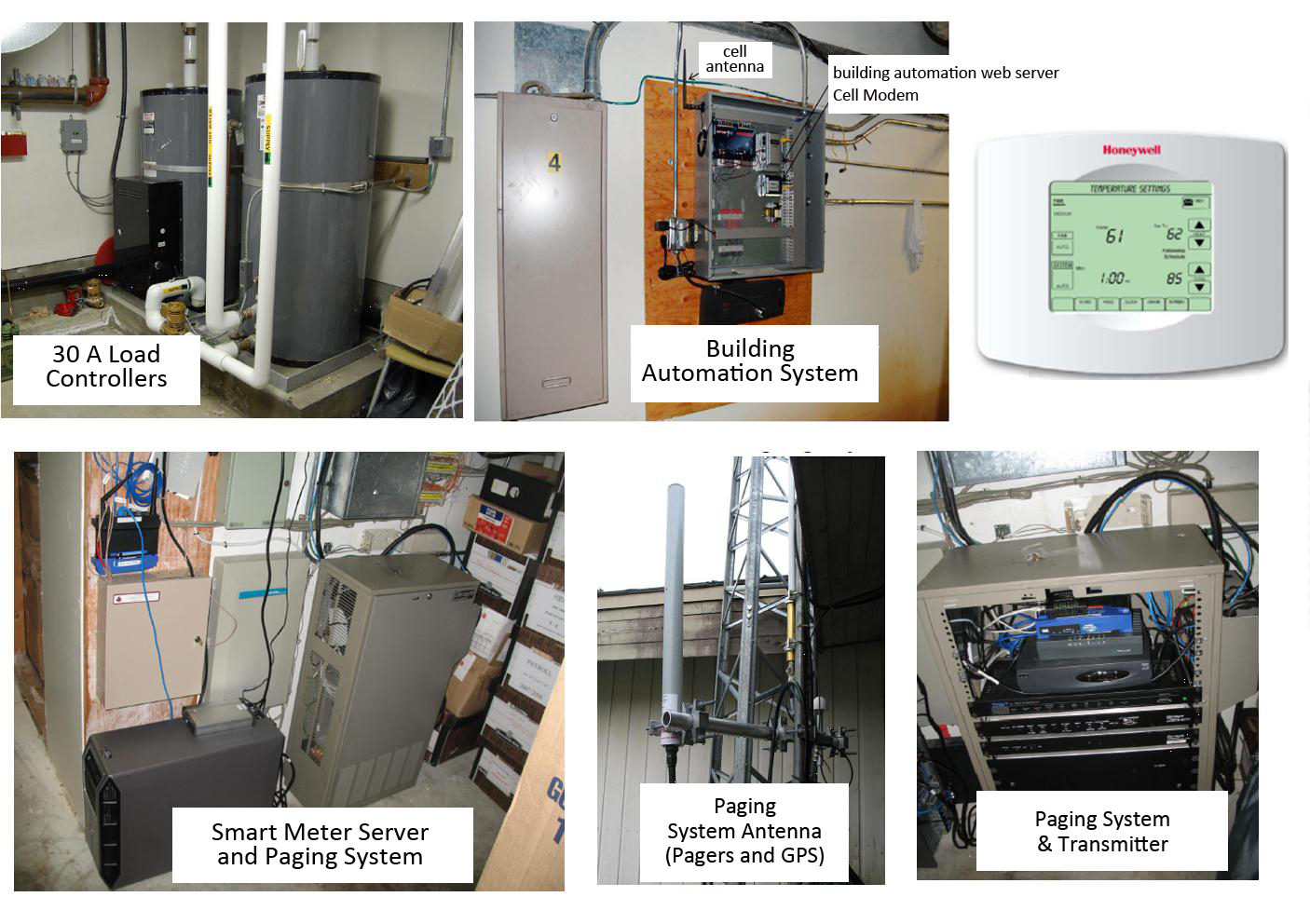
Figure 5: DR system components (Credit: Pulse Energy).
Text version
DR system components (Credit: Pulse Energy).
This figure shows a series of pictures illustrating the demand response system components. The first picture shows two 30 A load controllers. Next are a building automation system and an electronic thermostat. We can then see a smart meter server and paging system. The last two pictures show a paging system antenna outside (pagers and GPS) and a paging system and transmitter.
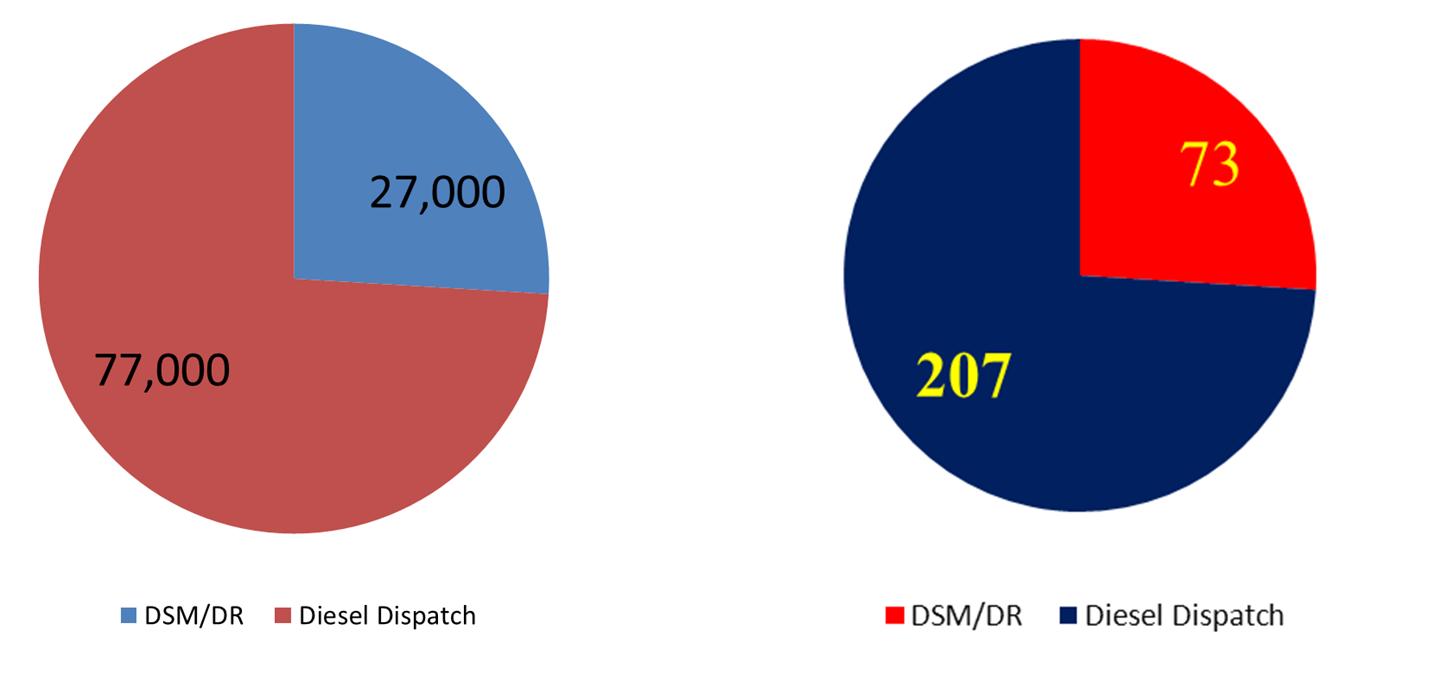
Figure 6: Estimated Savings in Diesel Consumption (litres per year, Left) and GHG emissions (tonnes per year, Right).
Text version
Estimated Savings in Diesel Consumption (litres per year, Left) and GHG emissions (tonnes per year)
Estimated savings in diesel consumption are 27,000 litres per year for the demand side management/ demand response system and 77,000 litres per year for the diesel dispatch. Moreover, estimated reduction in greenhouse gas emissions are 73 tonnes per year for the demand side management/demand response system and 207 tonnes per year for the diesel dispatch.
Project Team
CanmetENERGY: Tarek EL-Fouly (project manager), Lisa Dignard-Bailey and Steven Wong
Village of Hartley Bay, BC: David Benton (local champion)
Pulse Energy, BC: Bruce Cullen, Greg Dennis and Michael Wrinch
BC Government: Christina Ianniciello and Andrew Pape-Salmon
References & Publications
- Michael Wrinch, Greg Dennis, Tarek H.M. EL-Fouly and Steven Wong, “Demand Response Implementation for Improved System Efficiency in Remote Communities - Pilot Results from the Village of Hartley Bay”. IEEE Electrical Power and Energy Conference (EPEC 2012), London, Ontario, Canada (October 10-12, 2012)
- “Hartley Bay Micro Smart Grid: Demand Response Performance Analysis”. Technical report prepared by Pulse Energy (March 28, 2012).
- Michael Wrinch, Tarek H.M. EL-Fouly and Steven Wong, “Demand Response Implementation for Remote Communities - Installation Challenges and Initial Results for the Village of Hartley Bay”, IEEE Electric Power and Energy Conference (EPEC) 2011, Winnipeg, Manitoba, Canada. (October 3-5, 2011).
- “Hartley Bay Micro Smart Grid: One Year System Performance and Benefit analysis”. Client technical report prepared by Pulse Energy (July 31, 2011).
Page details
- Date modified: