Sensitivities and Adaptation of Ecosystems and Sectors
3.1 TERRESTRIAL ECOSYSTEMS
3.1.1 Sensitivities
Most natural systems have moderate to high exposure to the impacts of climate change, when considered over a time period of approximately 50 years. Because natural systems have evolved in response to climate since deglaciation, a sudden shift in climate conditions will challenge their ability to adapt. Boundary shifts at the ecoregion and ultimately the ecozone level may be expected. Climate change will lead to shifts in seasonally dependent biological rhythms and cycles, loss of habitat, extirpation or extinction of native plant and animal species, and the arrival of more invasive species. Other anthropogenic factors, principally those related to land use, will compound these impacts of climate change.
Changes in ecosystems and dominant species will occur due to changes in climate, either through conversion (replacement of the dominant species by a subdominant species) or migration (long-distance movement of species that can rapidly adapt to new soil or topographic factors; Nielson et al., 2005). In southern Atlantic Canada, the future fate of the already highly stressed ecosystems of the remnant Acadian Forest (Figure 12) remains uncertain (Mosseler et al., 2003a, b; Moola and Vasseur, 2004). The limit of the northern boreal forest may expand at the expense of tundra areas (Heal, 2001), although topographic and soil factors will limit the migration of treeline ecosystems (Holtmeier and Broll, 2005; Nielson et al., 2005). Available evidence suggests little or no recent observed change in the northern treeline position in Canada (Masek, 2001).
In concert with the regional warming in spring and summer, there has been a 5 to 6 day advance since approximately 1959 in the onset of phenological spring in eastern North America, as indicated by earlier leaf appearance or flower blooming and earlier bird nesting times (Schwartz and Reiter, 2000). In Atlantic Canada, earlier phenology of spring bloom was observed in interior regions, such as the Annapolis Valley (NS), but no significant differences were seen along the coast (Vasseur et al., 2001). Frequent episodes of winter thaw and late spring frost have led to widespread tree crown dieback in yellow birch throughout eastern Canada (Cox and Arp, 2001; Bourque et al., 2005; Campbell et al., 2005). Over the next few decades, shifting phenology of biological events may be either positive (e.g. enhanced productivity for trees) or negative (e.g. in the case of winter thaw, leading to trunk cracking for red spruce; see Mosseler et al., 2000). Birds are subject to problems associated with climate change, especially in relation to changes in the phenological spring. Early onset of warm days during the nesting season has been shown to negatively affect the breeding success of nesting seabirds due to heat stress and mosquito parasitism (Gaston et al., 2002). The timing of bird migration is also affected by increases in spring temperature, although long-distance migratory birds appear to alter the timing of migration in response to changes in weather and phenology (Marra et al., 2006). The broad spectrum of bird life in a region represents many different habits and habitats. Because they are widely observed and studied, they are useful indicators of environmental change (Boucher and Diamond, 2001).
Long-distance migration requires energy, and migrating birds must balance obtaining food with travelling in less-than-ideal weather. Use of a particular stopover varies with local weather and climate, and shows large interannual variation. The linkages to environmental factors suggest that migrating birds are sensitive to climate variability, which has implications for conservation efforts. A detailed study of the impacts of climate change on migratory songbird species in Atlantic Canada is in progress (Taylor, 2006).
Wildlife population dynamics are closely linked to climate. The seasonal migration of white-tailed deer in New Brunswick appears to be conditioned by winter climate variability as it affects snow cover (Sabine and Morrison, 2002). Milder winters may see this species occupying areas where they are currently absent in winter. Moose are at their southern limit of distribution in Nova Scotia and, under a warming climate, are expected to disperse northward (Snaith and Beazley, 2004). A review of NAO-related effects on northern ungulates found declines in caribou populations in northern Quebec and Greenland during periodsof warmer winters (Post and Stenseth, 1999), suggesting that woodland caribou populations of Newfoundland and Labrador will be negatively affected by climate warming.
3.1.2 Adaptation
Natural systems have proven to be relatively resilient in the face of previous climate changes. However, these changes occurred over longer periods of time and were not compounded by additional stresses imposed by humans. Left to themselves, ecosystems would evolve in response to changing environmental conditions. The necessity of using natural resources for human purposes, however, means that short-term changes in ecosystems are cause for human concern.
Differences in lifespan and size of individual organisms influence the degree to which each species is exposed, as well as the immediacy of the reaction to changed conditions. Insect species respond more rapidly to climate variation and change, in terms of both survival and migration, than do trees and large mammals.
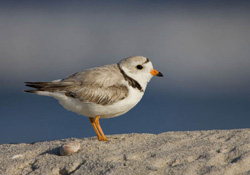
FIGURE 13: Piping plover, an endangered species that utilizes coastal areas. Photo courtesy of Sidney Maddock.
Approaches for adaptive responses to climate change impacts on natural ecosystems include comprehensive, integrated land-use management combined with protecting key habitats and species, promoting sustainable use of plant and animal species, and mechanisms for public education, awareness and action (Gitay et al., 2001; MacIver and Wheaton, 2005). Although comprehensive regional planning for biodiversity protection is yet to be realized for Atlantic Canada, all provinces have some kind of protected areas strategy, as well as wildlife and forestry management policies and legislation, and there are equivalent structures in areas of federal jurisdiction. For species at risk, such as the St. Lawrence aster and the piping plover (Figure 13) along the southern Gulf of St. Lawrence, and Long ’s and Fernald’s braya in northern Newfoundland (see Box 3), it is important that climate change sensitivity and risk analysis are considered in recovery management plans. Maintaining and enhancing the interconnected network of parks and protected areas is one means of enhancing the ability of natural ecosystems to adapt to changing conditions (Mosseler et al., 2003a, b; Beazley et al., 2005). Although protected areas are themselves subject to the effects of climate change (Scott et al., 2002), they can provide a base for monitoring and assessing ecosystem change that is less disturbed by human activity than their surroundings.
BOX 3 - Adaptive strategy for endemic limestone plants in Newfoundland
Plant and animal species are becoming extinct worldwide at an estimated rate of more than 20 per day, mainly due to habitat loss as a result of human activities. Recognizing the importance of maintaining biodiversity, governments have enacted legislation to recognize endangered species and facilitate their survival and restoration (Environment Canada, 2003). Climate change compounds the problem of critical habitat protection and species recovery.
Among species listed as ‘endangered’ is the arctic-alpine plant, Long’s braya (Braya longii). It is found only in Newfoundland and occurs only on limited coastal limestone barrens near the tip of the Northern Peninsula. A second species, Fernald ’s braya (B. fernaldii), listed as ‘threatened,’ has a slightly wider and overlapping distribution in the same area. Together with the endangered barrens willow (Salix jejuna), these species are endemics that are found globally only on these limestone barrens. Hybrids between the two braya species have become established, primarily where road construction and gravel removal have allowed close contact. Both braya species are subject to infection by a fungal pathogen and suffer predation from larvae of the diamondback moth (a species that migrates to Newfoundland in spring and summer). The steep latitudinal gradient of temperature northward along the peninsula sets a limit for the severity of these effects. As several recent warm years have indicated, an increase in regional temperatures, as projected in climate change scenarios, will very likely intensify these natural threats (Hermanutz et al., 2004; Parsons and Hermanutz, 2006).
As with other species at risk in Canada, a recovery strategy has been implemented for the braya and barrens willow populations of the Northern Peninsula that combines habitat protection, monitoring and research. Given the importance of human disturbance, community stewardship is critical, and a strong sense of responsibility is evident in the communities. In the long run, climate change may mean Long ’s braya does not survive in its present setting. However, the adaptive strategy in place with the Limestone Barrens Species at Risk Recovery Team will provide for preservation of the species in the provincial Botanical Gardens (Hermanutz et al., 2002).
3.2 COASTAL ZONE
3.2.1 Sensitivities
Atlantic Canada is defined by its coastal environment. With increasing pressure from coastal development (mostly housing development and community wharves) and sea-level rise, erosion and flooding are increasing and will continue to increase in frequency (Daigle et al., 2006). Consideration of geological factors, rate of sea-level rise, amounts of coastal erosion, wave climate and tidal regime allow calculation of the sensitivity to sea-level rise of shoreline segments (e.g. Gornitz et al., 1993). This assessment has been completed for all of Atlantic Canada on a broad regional scale (Figure 9; Shaw et al., 1998), and more detailed assessments have been conducted for specific segments of the coastline (e.g. Chmura et al., 2001; Catto et al., 2003; Daigle et al., 2006; Shaw, 2006). Erosion occurs on the most sensitive coastlines, such as sand dunes, sand and pebble gravel beaches, or where unconsolidated sediments or weakly consolidated bedrock form coastal bluffs. Dune-backed coasts are present in all four Atlantic provinces.
Throughout the southern Gulf of St. Lawrence, the combination of rising sea levels, increased human utilization of the coast for residential and tourism purposes, and limited offshore winter ice conditions have resulted in accelerated erosion and degradation of the dunes and coastline, such as in northeastern Prince Edward Island (Catto et al., 2002), southwestern and western Newfoundland (Pittman and Catto, 2001; Catto, 2002; Ingram 2005) and eastern Newfoundland (Catto, 1994). Winter storm erosion tends to result in coarser beaches with steeper profiles. Local factors, however, play a dominant role in the results observed at any particular beach (Catto et al., 2003; Catto, 2006a, b, in press).
Other coastlines are also sensitive to erosion (Figure 14 a, b). Coastal erosion rates in excess of 5 m/year have been measured in bluffs composed of glacial sediment at Chezzetcook, NS, coupled with landward migration of barrier beaches (Forbes et al., 1995; Orford et al., 1995; Taylor et al., 1997). Erosion rates of 0.7 m/month between December 2003 and April 2004 were observed at Sandbanks Provincial Park, NL (Ingram, 2004). Erosion at Cascumpec Bay, PE between 1974 and 2004 caused coastal retreat of 115 m, a rate of 3.8 m/year (Conroy, 2007).
Ongoing sea-level rise is increasing the risks associated with storm activity (Taylor et al., 1996a; Shaw et al., 1998, 2001; Bruce et al., 2000; Parkes et al., 2006). Coastal erosion, accelerated by rising sea levels, has occurred at several localities, notably along the south coast of Nova Scotia (Taylor et al., 1985, 1996a; Shaw et al., 1993, 1994), in eastern New Brunswick (Ollerhead and Davidson-Arnott, 1995; Shaw et al., 1998; Daigle et al., 2006; Ollerhead, 2006), along the north coast of Prince Edward Island (Forbes et al., 2002; McCulloch et al., 2002) and along Conception Bay, NL (Taylor, 1994; Liverman et al., 1994a, b; Batterson et al., 1999; Catto et al., 2003). As an example, observations at Mobile, NL between 1989 and 2005 (Catto, 2006 b) indicate that erosion has increased in the upper part of the beach system. Insufficient compensating deposition has occurred in the lower areas to maintain the overall volume of sediment. This beach is becoming coarser and narrower as sea level rises, and apparently less stable as storm activity, notably in winter and spring, combined with human foot traffic, results in enhanced profile modification.
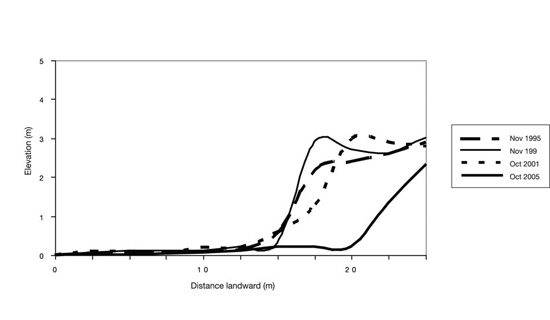
FIGURE 15: Successive measurements along a beach transect, Mobile, NL, showing erosion between November 1995 and October 2005 (Catto, in press).
An example of detailed mapping and evaluation of the sensitivity of a coastline to erosion is provided for Conception Bay South and Holyrood. This coastal sensitivity map enabled the recognition of four main potential hazards: coastal flooding and storm surges, damage to coastal infrastructure by storms, coastal erosion and damage to the ecology of coastal areas (Shaw et al., 1998; Catto et al., 2003).
3.2.2 Adaptation
There are three generic categories of adaptation that can be used in areas affected by coastal erosion, sea-level rise and/or storm-surge activity (Nicholls and Mimura, 1998; Nicholls, 2003):
- Planned retreat involves recognition of the inevitability of coastal erosion, and responds by abandoning the areas closest to the shoreline or locating only temporary or expendable structures in these areas.
- Accommodation involves constructing structures in ways that minimize damage (e.g. by placing buildings on elevated pylons) or developing land-use and zoning plans that allow only those structures that must be built on the shoreline (e.g. port facilities or fish-processing plants) to be located there, while prohibiting others (such as private residences).
- Protection involves physical reinforcement of the shoreline, either by ‘hard’ measures (seawalls, rip-rap, groynes) or by ‘soft’ measures (e.g. vegetating dunes with marram grass).
Municipal planning that integrates a combination of the three categories can lead to long-term solutions for communities.
The simplest way to accommodate a major hazard is planned retreat. This involves designating a building set-back limit and establishing a zone along the coastline where no permanent construction is permitted. In the case of Shediac, NB, analyses of the historical damage and the current trends due to sea-level rise, storm surges and coastal erosion led to discussion of possible adaptation, including retreat. Discussions were based on susceptibility of the residences in terms of economic compensation related to flooding, and included consideration of flooding area classes 5 and 6 (the most exposed zones). In New Brunswick, some planned retreat would be effective through the Coastal Areas Protection Policy (New Brunswick Department of Environment and Local Government, 2002) that is being implemented. This policy also encourages protection of the coastal areas through the avoidance of construction within 30 m of the high-tide shore, with permanent structures only permitted outside the set-back limit.
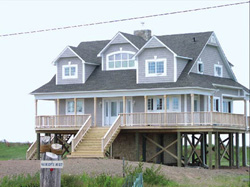
FIGURE 18: Construction of buildings on pylons as an example of adaptation that reduces vulnerability to storm surges, Grand-Barachois (near Shediac), Northumberland Strait, southeastern New Brunswick. Photo courtesy of Armand Robichaud.
The New Brunswick Coastal Areas Protection Policy (New Brunswick Department of Environment and Local Government, 2002) provides an umbrella for coastal zone management and adaptation measures at a local level. However, stakeholders and planners indicated that delay in implementation of the policy created a rush of building near the shore, with owners wanting to build before regulations were enacted (Martin and Chouinard, 2005). Despite the risks involved, many are still willing to build near the shore. Although there are tools available and municipality representatives should have local plans that can be used to control development in coastal areas of their community, there is a perceived lack of resources and consistency of application (Martin and Chouinard, 2005), leading to complaints from individuals, municipality representatives and environmental groups. Studies in Prince Edward Island, New Brunswick and Newfoundland have underlined the need for better planning in rural and urban areas that includes sea-level rise and stormsurge considerations (e.g. Paone et al., 2003; Smith et al., 2005). However, there are currently no coastal protection policies in Prince Edward Island, Newfoundland or Nova Scotia (with the exception of the Beaches Act), and some areas are facing significant pressure from development. In coastal regions, attachment to the area is strong and few individuals have considered retreat as an option. A long-term erosion rate is a useful guide for the establishment of set-back limits (Taylor, 1994) and indicates where specific structures are in danger. The absence of long-term monitoring of coastal erosion, however, means that present erosion rates may not serve to indicate the magnitude of previous (or future) events. In addition, as the majority of the erosion is caused by individual storms, hazard assessment requires consideration of the probability of the maximum impact of a particular storm, rather than only monitoring and dealing with the small, incremental removal of sediment on a daily basis. Accommodation can allow impacts on natural systems to occur while adverse impacts on people are minimized by adjusting the human use of the coastal zone. At present, accommodation is not commonly employed as an adaptive strategy in Atlantic Canada. Nevertheless, there are local examples of houses constructed on elevated pylons (Figure 18), allowing storm-surge waters to pass beneath, in coastal New Brunswick and Nova Scotia, that have been constructed as a result of decisions taken by individual homeowners. Comprehensive zoning has been suggested as an option in many areas (e.g. New Brunswick Department of Environment and Local Government, 2002), but deviations and variances are commonly granted.
Protection, in which the effects of extreme events, ongoing erosion and climate-related changes to natural systems are controlled by soft or hard engineering, is frequently used as an adaptive strategy. Seawalls, breakwaters and groynes, and emplacement of rip-rap and gabions, are the most common adaptive measures and those most favoured by the majority of coastal residents and property owners. Such hard engineering structures are expensive, require constant maintenance and observation, and can fail if not adequately designed and constructed. Repetitive storm activity and rising sea level both pose problems for the design and maintenance of hard coastal protection. In addition, some communities and land owners have used maladaptive techniques to protect their coastal properties, such as using different construction materials in levees and transition sections of levees, leading to inconsistencies (as occurred in New Orleans; see Nicholson, 2005).
In some areas, such as the Victoria Park area of Charlottetown, PE, aesthetic concerns influence the design of shoreline protection. In Summerside, PE and Trout River, NL, protective structures were designed to also provide a recreational walking trail. In some jurisdictions, protective measures are integrated in legislation, such as the Nova Scotia Beaches Act (Nova Scotia House of Assembly, 2000).
Soft engineering options include grading coastal bluffs to reduce erosion and planting or maintaining existing vegetation. Use of marram grass for this purpose is common in coastal dune areas in Atlantic Canada (e.g. Irving Eco-Centre in Bouctouche, NB; G. Arsenault, pers. comm., 2004). Conifers are also used, although they are more expensive and subject to damage from salt spray. Restoration of salt marshes is also potentially effective as an adaptive response to protect coastlines from rising sea level (Ollerhead, 2006).
An important aspect of any adaptation strategy is development of an understanding amongst the residents of the key issues facing their community. Community-based planning and activities are likely to be most effective. In order to involve the community, an education and public awareness program would need to be planned and implemented. Without community support, neither planned retreat nor accommodation through zoning can be effectively implemented.
Although most provincial legislation and municipal development plans (e.g. Beaubassin Development Commission; Daigle et al., 2006) have provisions to protect coastal zones, very few integrate climate change in their long-term planning and protection of these habitats, leading to difficulties in adapting to changes in sea level and coastal erosion. As a result, coastal infrastructure may have to be moved or face the risk of damage over time. In most cases, the lack of long-term planning, funding and available land to which to move the infrastructure are limiting factors for adaptation (DeLusca et al., 2006).
3.3 MARINE ECOSYSTEMS AND FISHERIES
3.3.1 Sensitivities
Marine resources form a vital socioeconomic component throughout Atlantic Canada. Direct impacts of climate change on biological species result from changes in surface and near-surface ocean temperatures, changes in sea-ice duration and extent, and changes in nearshore and beach areas used by species for feeding and spawning. Changes in pest species and pathogen distribution pose additional concerns. Aquaculture operations could be impacted by changes in storm activity along coastlines, coastal erosion of protective salt marshes and barrier dune systems (particularly along the Gulf of St. Lawrence coast), and changes in freshwater and terrestrial sediment influx into estuaries. Climate change would also affect numerous aspects of fishery operations, including transportation, marketing, occupational health and safety, and community well-being (see also section 3.10; Catto et al., 2006; Catto, in press; Sjare et al., 2006). Many of these impacts are related to changes in sea state and storm activity.
The role of climate change with respect to fisheries, fish harvesters and fishing communities has varied throughout the northwestern North Atlantic, both by place and over time, from that of ‘supporting player’ to mere ‘background noise’ (Catto and Catto, 2004). Only in cases of collapse of fish stocks due to purely ecological causes could climate change be considered as the ‘driving force’.
The fisheries of Atlantic Canada are influenced by the Gulf Stream and the Labrador Current. The Gulf Stream is the most defined and strongest current in the North Atlantic, carrying 55 million m 3/second of water along the Atlantic Canadian seaboard (Narayanan, 1994; Beer, 1996; Kearns, 1996). Anticipated future conditions involve warming of the Gulf Stream and reduced influx and warmer water from the St. Lawrence River, particularly during the summer months. Increased southwesterly wind activity would produce an increase in current flow towards Atlantic Canada. In the Gulf of St. Lawrence, reduced and warmer flow from the St. Lawrence River during the summer would weaken the cold countercurrent that flanks the east coast of New Brunswick, all coasts of Prince Edward Island and the Atlantic coastline of Nova Scotia, generating further summer warming along these shores. The simultaneous weakening of the countercurrent and warming of the Gulf Stream would provide suitable conditions for the northward spread of marine organisms from the mid-Atlantic coastline of the United States, including both economically useful fish species and pests and pathogens.
Warmer water conditions in the Gulf Stream would result in lengthening of the ‘soft-shell’ phase of crab moulting. During this time, harvested crab cannot be effectively processed or marketed, leading to wasted effort and loss of income for harvesters. Fish harvesters have noted difficulties with earlier development of ‘soft shell’ in recent years. Early juvenile crab appear to be the most sensitive to changes in water temperature, since they have very narrow habitat requirements; this creates a weak link in the life cycle of the snow crab (Dionne et al., 2006).
In coastal waters of New Brunswick, Nova Scotia and Prince Edward Island (including those used for aquaculture), summer decreases in rainfall over land would result in diminished flow of river systems. In estuarine environments, low-flow events during the summer currently result in enhanced intrusions of saltwater wedges, thus raising salinity. Lowered velocity and reduced influx of rivers facilitates the spread of sea lettuce (Ulva lattuca), which acts to increase eutrophication, rendering estuaries both less suitable for shellfish or finfish aquaculture and less attractive to residents and tourists (Figure 21). The increased extent of sea lettuce in Cascumpec and Tracadie bays observed between 1990 and 2006 is the result of diminished freshwater influx, reduced circulation of the estuaries and addition of fertilizers from agricultural areas (Conroy, 2007). Changes in agricultural practice that entail more use of irrigation and fertilizer will increase the likelihood of sea lettuce expansion. Reduced influx from the Saint John (see Bruce et al., 2003), Petitcodiac and Annapolis rivers would produce similar effects in Bay of Fundy coastal waters.
The Labrador Current is fed by freshwater influx from Greenland, Arctic Canada and Labrador, in addition to water from the European Arctic. An increase in the rate of glacial melting in Greenland would cause the Labrador Current to freshen and strengthen, bringing colder water and icebergs south along the northeastern Newfoundland Shelf (Canadian Institute for Climate Studies, 1999 –2005; Hadley Centre, 2006). Influx of colder Labrador Current waters locally produces fish kills, as in Smith Sound in February 2003 (Colbourne et al., 2003). Groundfish species such as Gadus morhua (cod) suffer terminal kidney failure when immersed in water temperatures below 2°C and react adversely to rapid decreases in temperature (Clark and Green, 1991; Chabot and Dutil, 1999; Petersen and Steffensen, 2003; Mosaker, 2005). Range contraction of cod in response to colder nearshore waters is well documented along the west coast of Greenland (e.g. Hansen, 1949). Strengthening of the Labrador Current would increase the probability of similar events in future. Changes in the strength of the NAO would also change water temperatures, thus impacting fish populations, notably cod (Drinkwater et al., 2003).
Changes in cod stocks are also related to the capelin (Mallotus villosus) population, a preferred prey species. Capelin is also a major food source for seabirds (Davoren and Montevecchi, 2003; Davoren et al., 2003) and is one of the most important species in the Atlantic region, ecologically and economically. Capelin stocks are concentrated in the region extending from the 2 °C isotherm southwards to the northern fringes of the Gulf Stream (Narayanan et al., 1995). Spawning occurs both offshore and in nearshore and bea ch zones around Newfoundland, and capelin spawning time is inversely correlated to the temperature of the uppermost 20 m of the marine water column (Narayanan et al., 1995; Carscadden and Nakashima, 1997; Carscadden et al., 2000). Colder water conditions induce spawning later in the season. The health of capelin stocks is thus a critical indicator of the impact of changes in water temperature (Rose, 2005). Strengthening of the Labrador Current with cold, fresh water derived from melting glaciers in Greenland, combined with northeasterly winds pushing colder waters onshore, could thus negatively impact capelin, leading to later spawning times. If spawning is delayed until the start of hurricane activity, the net result could be a loss of productivity.
Changes in the extent and timing of sea-ice formation could impact seals and other marine mammals (Sjare et al., 2006). In several Newfoundland river and estuarine systems, seals have increased residence times by up to 3 months since the 1990s (Lenky et al., 2006). Historically, seals were not present in estuaries during the salmon smolt run. The increased time spent in estuaries by seals could lead to increased predation on both capelin and salmon. Further research is ongoing to assess the effects of seal predation on salmon stocks (Lenky et al., 2006).
The impacts of climate change are substantially different on nearshore fisheries and aquaculture in Labrador and northeastern Newfoundland compared to Maritime Canada. In the Maritimes and southwestern Newfoundland, summer impacts are primarily related to warming and increased salinity, whereas cooler waters (particularly during winter) will be notable in northeastern Newfoundland embayments. In Placentia and St. Mary ’s Bay, where the Gulf Stream and the Labrador Current seasonally interact, the transition between winter (Labrador Current –dominated) and summer (Gulf Stream–dominated) conditions would be expected to become sharper, having adverse impacts on fish species (e.g. Rose, 2005).
Most freshwater and marine aquatic species are adapted to a relatively narrow range of temperature conditions. With warmer water temperatures, salmonid species in Atlantic Canada will most likely suffer range contraction or extirpation as a result of changing habitat, introduced competitors and predators, and increased parasitism (Marcogliese, 2001; Schindler, 2001; El-Jabi, 2002). Significant changes have already been recorded in some rivers, such as the Miramichi in New Brunswick, and are correlated with loss of production due to unsuitable conditions for growth and survival of juvenile Atlantic salmon (Swansburg et al., 2002; El-Jabi and Swansburg, 2004).
Changes to river ice activity, either increases or decreases, would alter the overall ecological character of a river. Adverse effects of enhanced ice scour activity on the salmonid populations of the Miramichi were noted by Beltaos and Burrell (2003). However, a reduction in ice scour can be equally detrimental. Depressions created by ice scour and slab wallowing form low-energy pools, which are utilized as resting areas by salmonids during summers. Ice scour also removes vegetation and muddy organic sediments from the banks, opening the shoreline and increasing accessibility for fauna (see Johnston, 1993). Driftwood transported by ice and the spring freshet provides important habitats for invertebrates, vital as food sources for salmonids as well as for pollination of key species such as blueberries (Harmon et al., 1986; Lomond, 1997; Colbo et al., 1999).
3.3.2 Adaptation
Harvesters of wild marine resources are constrained in their potential responses to climate change and variation by existing regulatory regimes. Whereas farmers can plant different crops or adjust harvesting times in response to climate variations or meteorological events, fish harvesters cannot choose to harvest a different marine species (without previously obtaining a specific license), fish at different times (i.e. outside the established season) or harvest amounts in excess of established quotas. The ‘soft-shell crab’ problem represents one example where the constraints of an established season may conflict with ideal harvesting time under the influence of higher Gulf Stream temperature. Although species licenses, quota systems and harvesting seasons must be maintained for effective fisheries management, adjustments on regional bases may be required to effectively manage harvesting under changing water temperature and sea-ice regimes.
Aquaculture operations are theoretically able to respond to climate shifts with more flexibility than harvesters of wild marine resources. Adaptation would be most effective if aquaculture operations were sufficiently flexible to allow shifts in the species cultivated and the periods of cultivation.
Adaptation will also be required to help Atlantic fisheries deal with operational and occupational health-and-safety concerns related to increased storms, storm surges and sea-level rise. The need for vessels to travel farther seaward to find suitable resources places ships and crews at greater risk in the event of increased storm activity. This results in more demand being placed on search-and-rescue operations, notably the Coast Guard. This problem is compounded by current federal regulations that specify vessel length without corresponding restrictions on beam and height, resulting in the design of top-heavy vessels susceptible to rollover in more extreme storm conditions. Vessel development and design is currently under review in several provinces (e.g. Canadian Centre for Fisheries Innovation, 2004; Newfoundland and Labrador Fisheries and Aquaculture, 2006), and further research would be valuable.
3.4 WATER
Freshwater resources in the four Atlantic provinces represent less than 4% of the total available fresh water in Canada. The province of Newfoundland and Labrador accounts for almost 90% of the total resource in Atlantic Canada (Natural Resources Canada, 2006a, b), with most of the resource located in Labrador. The largest watersheds are those of the Churchill River (79 800 km 2) and the Saint John River (35 500 km2 within Canada). Changes in temperature and precipitation influence evaporation and runoff, and the amount of water stored in glaciers, lakes, wetlands and groundwater (Freeze and Cherry, 1979; Jones, 1997; Hornberger et al., 1998: Rivard et al., 2003). This, in turn, results in changes in the quantity and quality of water available for human use, and impacts ecosystems and habitat.
Changes in water resources can have far reaching effects. Possible reductions in summer stream flow predicted for Atlantic Canada could impact tourism and recreation, freshwater fisheries, hydroelectric power generation, municipal water supplies and agriculture. Effects, however, will differ throughout the region. In some instances, the existing variability in annual and seasonal precipitation is greater than the anticipated impact of climate change (e.g. Barnard and Richter, 2004).
3.4.1 Sensitivities
Surface Water
Climate projections indicate that overall precipitation throughout most of Atlantic Canada, with the possible exception of western and central Labrador, will continue to increase (Figure 5b). However, increased precipitation does not necessarily lead to more water in rivers, lakes and wetlands. With increased summer temperatures, the increased rate of evaporation may exceed the influx of precipitation, causing declines in water levels. Global stream flow trends suggest that flows will increase in Labrador but decrease throughout the remainder of Atlantic Canada (Milly et al., 2005). Declining flows have been noted over the period 1970 to 2000 in the Saint John and St. Croix Rivers of New Brunswick (Bruce et al., 2003).
The increase in precipitation noted since 1948 (Lines et al., 2003) is not distributed evenly throughout the seasons in most areas of Atlantic Canada. Declines in summer precipitation at several Newfoundland sites (Catto and Hickman, 2004; Slaney, 2006) have contributed to seasonal desiccation of streams and wetlands, impacting salmonid populations (e.g. Marcogliese, 2001; Schindler, 2001) and causing shortages of domestic water supplies, as occurred in St. John ’s and other Newfoundland communities during the summers of 1997, 2003, 2004 and 2005.
Wetlands form a key element in the supply of water to stream systems, particularly during the drier summer months. Wetlands also moderate flooding by reducing influx to stream systems immediately following rainstorms (Freeze and Cherry, 1979; Jones, 1997; Hornberger et al., 1998). Overexploitation, inadequate wetland protection, and habitat destruction of wetland areas compound the problems generated by changes in seasonal rainfall (Schindler, 2001). In urban areas, development on former wetlands and floodplains can contribute both to lower water levels during summers and to flooding following rainstorms (e.g. Watt, 1989; Catto and St. Croix, 1998; Wohl, 2000; Liverman et al., 2006; Catto, 2006 b). Even in areas without substantial urban development, clearance of forests and drainage of wetlands have led to accentuated flood risk (e.g. Bosch and Hewlett, 1982).
Summer drought would likely diminish wetland areas. The effects would be felt primarily in areas that have been under stress from other causes. In communities where wetlands have not seen substantial development, decreases in summer rainfall within an overall pattern of increasing annual precipitation have not led to widespread desiccation (e.g. Slaney, 2006). Maintenance of wetlands is thus an effective mechanism for reducing the effects of seasonal variations in precipitation on stream flow.
Lowered water levels or decreased river flows could also lead to poor drinking water quality in some areas. Many municipalities throughout Atlantic Canada depend on surface water supplies, leaving them exposed to declines in water level in ponds and rivers, and contamination. A decrease in the supply of water to treatment plants could increase turbidity, resulting in the need for a greater level of treatment (Falkingham et al., 2001; Public Health Agency of Canada, 2002; Dolgonosov and Korchagin, 2005).
Increases in temperatures, prolonged summer dry seasons and heavier rainfall also increase the risk of contamination of drinking water by waterborne parasites, such as Giardia, Cryptosporidium and E. coli (Atherholt et al., 1998; Curriero et al., 2001; McMichael et al., 2003; Charron et al., 2004). Giardia cysts are frequently present even in treated water samples in Canada (Wallis et al., 1996), and are very common in raw sewage. In 2005, orders to boil water before human consumption were in place for more than 200 communities in Newfoundland and Labrador (Newfoundland and Labrador Environmental Industries Association, 2005). Similar ‘boil water orders’ have affected Charlottetown, Moncton and Saint John within the past 10 years.
Changes in snow cover will further impact water supply. The Maritime Provinces are expected to see a greater percentage of precipitation falling as rain rather than snow (Lines et al., 2003; Lines and Pancura, 2005). Winter runoff of rain means that less water would be available in the hinterland areas to replenish the lower reaches of stream systems during the summer (El-Jabi et al., 2004). Thus, an increase in winter precipitation would not result in an increase of available water for residents, unless winter runoff is stored in reservoirs. In Newfoundland and coastal Labrador, winter precipitation will increase, particularly during positive phase NAO winters. Winter thaw and rain events could lead to increases in rain-on-snow flooding, as has been seen in Corner Brook since 1950 (Catto and Hickman, 2004). The anticipated changes in precipitation will not produce significant effects in the wettest areas of the province, including the southwest coast and the Avalon Peninsula (Barnard and Richter, 2004), but study of other areas is required. In interior Labrador, reduced snow cover could impact hydroelectricity production, but increased precipitation in spring and fall would help maintain the Smallwood Reservoir, reducing the impact on the system as a whole.
Groundwater
Groundwater reserves in Atlantic Canada contain water from recent precipitation and from precipitation that fell decades to centuries ago. If water is withdrawn at rates in excess of recharge by precipitation, the result is a drop in the water table. For shallow wells in Atlantic Canada, lowered water tables result after periods of summer drought. Water tables in deeper wells drilled into bedrock generally respond to yearly to decadal variations in precipitation and withdrawal.
The areas of Atlantic Canada most dependent on groundwater are southern Nova Scotia, eastern New Brunswick and Prince Edward Island (Rivard et al., 2003). Reductions in the water tables would result from decreased influx of seasonal precipitation, due to a combination of reduced summer rainfall and enhanced surface runoff of winter rain. Higher temperatures and earlier phenological springs, leading to plant growth earlier in the year, would increase evapotranspiration, thereby reducing groundwater recharge.
Prince Edward Island relies almost entirely on groundwater (Rivard et al., 2003; Savard, 2006). Recharge zones along the heights of land are adjacent to the discharge zones in low-lying areas. Water tables are located near the surface, and groundwater is depleted following periods of summer drought (see Government of Prince Edward Island, no date). Winter snowfall is critical for recharge under these circumstances. Water shortages may be compounded if demand for water increases from urban Charlottetown, for seasonal tourism operations or for agriculture. Businesses and communities dependent upon summer tourism, in particular, have concerns. During the relatively dry summer of 2002, at least 145 tourism-related businesses on Prince Edward Island were required to institute boiling of water (Prince Edward Island Eco-Net, 2003), necessitating careful monitoring of water supply and preventive maintenance. Drawdown of the water table due to withdrawal for irrigation is presently confined mainly to the direct vicinity of the wells (e.g. Somers and Mutch, 1999), but increased demand for water from all users will increase pressure on the resource.
In coastal communities, drawdown of the water table adjacent to the ocean, in conjunction with sea-level rise, allows seawater intrusion inland, eventually contaminating wells and rendering the water undrinkable. The areas most susceptible are coastal plains, such as southeastern New Brunswick and much of Prince Edward Island (Scott and Suffling, 2000). Low-lying areas adjacent to the Bay of Fundy, such as Wolfville, NS and Moncton, NB, are also susceptible, as both areas also have increasing demand for fresh water from agriculture and housing developments (Boesch et al., 2000).
Rapid withdrawal of groundwater in coastal areas can result in saltwater intrusion within a relatively short period. At L ’Anse-aux-Meadows, with sea-level rise and the influx of approximately 35 000 tourists during the Viking Millennium celebration between mid-July and mid-August 2000, the drilled well at the Norstead re-enactment site experienced drawdown and salinization within one month (G. Noordhof, pers. comm., 2006). Ongoing salinization at L ’Anse-aux-Meadows has required the owner-operators of the only restaurant in the community to dig a new well in each year since 2002 in order to supply their business with fresh water, and has effectively precluded establishment of other tourist-related businesses at L ’Anse-aux-Meadows. In this case, the increase in tourist usage of groundwater has greatly accelerated the progress of salinization.
Flooding
Changes in the amount, timing and nature of precipitation can result in increased flooding frequency (Clair et al., 1998; Ashmore and Church, 2001). In the boreal climates of Atlantic Canada, winters with increased precipitation and marginal changes in temperature could result in an increase in rain-on-snow events that result in a large portion of the rain rapidly running off, rather than infiltrating the ground. Increased rain-on-snow flooding is already evident on the Saint John River (Beltaos, 1997; Catto, in press) and in the Corner Brook area (Catto and Hickman, 2004). In Nova Scotia, rain-on-snow events have led to more than 50 periods of severe floods since the beginning of records in 1759 (see Environment Canada, 2004a), including recent events in Colchester, Cumberland, Hants and Kings counties (e.g. Catto, in press). Mid-winter flooding during January 1956 resulted in the destruction of more than 100 bridges throughout Nova Scotia (Environment Canada, 2004a). A rain-on-snow flooding event that carved a 10 m deep channel through Bishops Falls, NL destroyed the Abitibi-Price powerhouse and dam, all major roadways and numerous structures, and required evacuation of the community (Ambler, 1985), costing more than $34 million (1983 values). In late winter 2006, rain-on-snow events impacted the Burin, Baie Verte (Middle Arm, Fleur-de-Lys, Baie Verte town) and northeastern Avalon peninsulas. Along the Miramichi River, threats to cemeteries and other historical sites would increase because several historical and small community sites are located along the shore. Flooding of cemeteries has also been noted in Newfoundland, at Appleton and Indian Bay (Catto and Hickman, 2004; Catto, in press).
The Atlantic provinces experience alternating cold and mild spells throughout the winter and early spring, resulting in several freeze-up and break-up events in rivers each year (Watt, 1989; Shabbar and Bonsal, 2003). The combination of generally thicker winter ice cover (Clair et al., 1997), combined with irregular warm periods, will increase the potential for dynamic ice jams and consequent flooding. Dynamic ice jams result from the deposition of floating ice against obstructions in the river channel (Beltaos, 1983; Beltaos and Burrell, 2003). The formation of a dynamic ice jam occurs when a solid ice cover, formed during a cold period, resists break-up when impacted by a high-velocity flow event. Significant ice jam floods have occurred in recent years at Badger, NL (Figure 22; Fenco Newfoundland Limited, 1985; Picco et al., 2003; Peddle, 2004) and Perth-Andover, NB (Beltaos and Burrell, 2003; Environment Canada, 2004a), and along the Miramichi River, NB (Beltaos and Burrell, 2003). If historical trends continue, ice break-up and flooding will become more frequent and unpredictable. This would result in property damage and the destruction of highways and bridges, and could affect hydroelectric power generation.
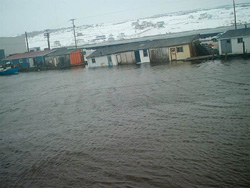
FIGURE 23: Flooding in St. Lawrence, NL., due to storm surge from Placentia Bay, February 2004, triggered by southwesterly wind (from Southern Gazette, Marystown, NL).
Flooding is also associated with hurricane and storm events (Figure 23). Mid-latitude storm systems have caused serious flooding in communities in Atlantic Canada, such as Stephenville and St. John ’s, NL (e.g. Liverman et al., 2006). Extreme rainfall events over hydroelectric reservoirs can induce flooding, if the volume of rainwater exceeds the capacity of the reservoirs. All of the major population centres of New Brunswick have previously experienced river flooding.
Regardless of the cause of a flood, human health can be negatively affected and serious injury or death may result. Drowning, hypothermia and electrocution are all risks directly associated with floods (Environment Canada, 2004d; Health Canada, 2005; Jonkman and Kelman, 2005). Contamination of floodwaters by sewage, wastes and domestic, industrial and agricultural chemicals is common, resulting in lengthy and expensive cleaning procedures and delay in reoccupation of communities (e.g. Peddle, 2004). Sewage systems and water treatment facilities may be overwhelmed with a sudden influx of contaminated floodwater. Submergence of buildings in floodwaters produces more favourable conditions for the growth of moulds and fungi that, if not removed, can lead to health difficulties when the buildings are reoccupied (Dales et al., 1991; Health Canada, 2005). Waterborne diseases and parasites can also be spread as a result of flooding (Curriero et al., 2001; McMichael et al., 2003; Charron et al., 2004). The chance of an outbreak of a waterborne illness is more than doubled during the six weeks following an extreme rainfall event (Thomas et al., 2005). Current water treatment practices cannot easily address these risks.
Much research on climate change and water resources has focused on physical aspects, with relatively less work done on the socioeconomic impacts. Social impacts are difficult to quantify, and therefore may not be recorded in monetary estimates of ‘total cost’ of flood damage (H. John Heinz III Centre for Science, Economics and the Environment, 2000; Mitchell, 2003; Parson et al., 2003). Social impacts may persist much longer than the physical impacts of flooding.
3.4.2 Adaptation
Adaptation to changes in water resources and flooding represents application of well-established ‘best practices’. All of the suggested adaptations would be advantageous to Atlantic Canada and its residents, regardless of climate change. Shortages in water resources for human consumption are best addressed through a combination of land-use and resource management, planning, and conservation and reduction of wasteful usage (Bruce et al., 2000). Preservation of wetlands has been documented as an effective adaptive response for runoff retention, both reducing spring flooding and increasing summer streamflow (see Watt, 1989; Booth, 2000; Coote and Gregorich, 2000; Wohl, 2000). Construction of retention ponds for storage of water and reduction of flooding, common in many areas of Ontario and western Canada (e.g. Ontario Ministry of the Environment, 1999; Marsalek et al., 2000; Environment Canada, 2004d; Kuehne and Cairns, no date), has not been common practice in Atlantic Canada. Adapting to changes in the frequency, intensity and duration of precipitation events will require adjusting reservoir management practices (c.f. Miller and Yates, 2006).
Urban municipalities in Atlantic Canada have been confronted with summer water shortages and water quality issues in recent years. Municipalities have responded by upgrading treatment facilities, accelerating development of new water supply areas, increasing security around surface water supplies and encouraging water conservation through combinations of educational programs and financial mechanisms. All of these adaptive mechanisms could be pursued further in the future.
Reliance on groundwater, particularly in coastal areas where saltwater intrusion due to rising sea level is coupled with decreased summer precipitation, necessitates conservation and careful monitoring of water quality. Detailed monitoring of consumption patterns, in combination with resource assessment, represents an effective adaptation approach, as in the Baltic, PE area (Somers and Mutch, 1999).
The most effective adaptation approaches to reduce flooding damage is to prevent new construction on flood plains, relocate existing structures and residents where economically feasible, and provide and maintain adequate drainage infrastructure for coping with rainfall events (Catto and Hickman, 2004). Relocation of structures and communities at risk has been successfully accomplished in several areas (e.g. Evans and Brooks, 1994; Shrubsole et al., 2003; Environment Canada, 2004d; Carter et al., no date), including Atlantic Canada (Peddle, 2004; Catto, 2006a). Mapping of flood-susceptible areas is a significant tool in reducing flood risk, when coupled with effective land-use planning and zoning. This requires effective communication between scientists, municipal planners, community leaders and residents (Berger, 2006; Leroy, 2006). Integrated mapping and assessment of all biophysical hazards affecting a community (e.g. Schmidt-Thom é et al., 2006a, b) is an effective starting point in the development of adaptive responses. Understanding the factors influencing the vulnerability of different communities to flood events is also important (see Box 4).
BOX 4 - Factors affecting community vulnerability to flooding
Communities throughout Atlantic Canada exhibit variability in sensitivity and vulnerability to flooding, in part because of differences in local awareness of the hazard and investment in effective adaptation. Key physical influences on vulnerability include the frequency and severity of flooding. Social and community influences on community vulnerability include such factors as population dynamics and demographics; the location of the residential developments and critical infrastructure in relation to flood-prone areas; monetary property values; degree of alteration of natural drainage routes by human activity; resiliency of transportation and communication routes; presence of hazard-limiting infrastructure; recovery and adaptation abilities; and the existence of emergency and development plans.
Social impacts may increase as a result of misconceptions regarding the frequency of a particular flood hazard. There is a tendency to overestimate the frequency of lower probability flooding mechanisms (e.g. landslides blocking rivers or tsunamis), and underestimate the frequency of higher probability flooding events, such as storm surges, rain-on-snow events and dynamic ice jams (Viscusi, 1993). Although repeated flooding from similar causes has occurred in some Atlantic Canadian communities (e.g. Liverman et al., 2006), individuals and communities may fail to take the necessary precautions, leading to financial losses, personal and societal stress, and further physical, financial and psychological damages.
Community vulnerability is partly a function of the distribution and number of higher risk groups, who tend to have fewer resources at their disposal to cope following an event. Studies conducted following disasters in Canada, the United States and Japan indicate that neighbourhoods and communities that were poor or declining socioeconomically before a disaster do not succeed in achieving the socioeconomic status that they possessed prior to the event, even as reconstruction efforts progress (Morrow, 1999; Morrow-Jones and Morrow-Jones, 1991).
The tendency for residents of rural areas and smaller communities in Atlantic Canada to remain in their communities for long periods, developing local ties, reduces vulnerability. For many communities in Atlantic Canada, however, declining and aging populations with decreasing economic resources exhibit greater vulnerability, not only to flooding but to all hazards, risks and changes.
3.5 FORESTRY
3.5.1 Sensitivities
In Atlantic Canada, increased growing season temperature and rising levels of CO2 will not necessarily result in increased ecosystem productivity (Flannigan et al., 2000). For example, for black spruce (Picea mariana), the dominant tree species in the boreal forest of Labrador, the net ecosystem productivity of the forest will likely increase with increased springtime temperatures. Nevertheless, elevated summer temperatures will likely decrease net productivity because of higher rates of evapotranspiration.
With decreased summer precipitation (Lines et al., 2003) and higher temperatures, the possibility of drought disturbance rises in Atlantic Canada (McCurdy and Stewart, 2003). Shallow-rooting trees, such as hemlock and spruce, are much more sensitive to drought than those with deep root systems (Dale et al., 2001).
With increased drought, the probability of forest fires increases significantly and the duration of the fire season is extended. Analysis of paleoecological data and modelling under General Circulation Model scenarios suggests a reduction of the potential for wildfires in eastern Ontario and Quebec, but an increase in Atlantic Canada, including Newfoundland and most of Labrador (Flannigan et al., 2001). Changes in forest fire characteristics have the potential for drastically altering interior forests by changing nutrient cycling. Forest fires also have implications for the health of nearby residents, with smoke and particulates aggravating respiratory illnesses (University of Washington, 2001; McMichael et al., 2003; University of British Columbia Okanagan, 2005; Moore et al., 2006).
Fire disturbance in ocean-influenced forest ecosystems has been extremely rare, with most disturbances originating from wind and storms (Figure 24; Wein and Moore, 1979; Runkle, 1985; Seymour, 1992; Foster et al., 1998). Increases in the strength and frequency of windstorms and thunderstorms are an important concern for forests. Wind speed is the determining factor in the extent of damage to trees (Figure 25). Larger trees are more susceptible to windthrow (McCurdy and Stewart, 2003), as are stands that have been recently burned (Flannigan et al., 2000). Damage to tree stands increases the probability of pathogen outbreak and tree mortality (Ayres and Lombardero, 2000).
Another aspect of climate change is the potential for an increased frequency of ice storms (Dale et al., 2001). Damage from ice storms, such as those that occurred in New Brunswick in 1998 (Natural Resources Canada, 2003b) and the Cobequid Hills of Nova Scotia in 2002 (Nova Scotia Department of Environment and Labour, 2003), can range from incidental damage of individual branches to annihilation of entire stands. Should a period of drought follow an ice storm, the probability of fire outbreak increases (Irland, 2000). Frequent episodes of winter thaw and late spring frost also pose problems, particularly for frost-sensitive species such as yellow birch (Cox and Arp, 2001; Bourque et al., 2005; Campbell et al., 2005).
Shifts in the abundance of insects, pathogens and herbivores have the greatest potential to adversely affect forests (Gray, 2005). Under a warmer climate, ranges of insects and pathogens are expected to shift north (Gray, 2005) and warmer winter temperatures will decrease mortality. With the relatively short lifespan of these species, the increased number of generations per season will allow for quicker adaptation to evolving climate conditions (Gray, 2005). As a result, greater numbers of insects and pathogens may disturb forest ecosystems, possibly resulting in changes to nutrient cycles and forest species composition that, in turn, can significantly alter soil associations for stands (Ayres and Lombardero, 2000). It is also possible that, in some regions, pathogen outbreaks may decrease if changed climate conditions favour the increased abundance of predators or competitors (McCurdy and Stewart, 2003).
Invasive species are also expected to benefit from a shifting climate, mainly due to their quick reproductive strategies that allow them to disperse quickly (Simberloff, 2000). Invasive species affect forests by shifting nutrient cycles, thus affecting forest succession and fire regime. Increases in the action of herbivores and predation can cause regional extinctions through hybridization with native species and cause increased mortality from the transportation of exotic diseases (Dale et al., 2001).
Climate change, and the broad nature of the associated disturbances that will accompany it, will have a tremendous impact on the genetic variation of forests. Genetic variation, the basis for forest health, will become increasingly important as climate shifts (Mosseler et al., 2003b).
3.5.2 Adaptation
Migration of tree species in response to changes in climate requires time, and there is a substantial time lag between the development of suitable climate conditions and the establishment of a forest. Considering the lifespan of tree species, adaptation options for the forest industry may be limited over the short term. Adaptation will be facilitated by management strategies that enhance the capacity of the forests to cope with shifting climate conditions and affected site conditions (Beaulieu and Rainville, 2005). Enhancement and preservation of genetic variability (e.g. during harvesting and reforestation) is critical, as it increases the ability of a forest stand to withstand an outbreak of pathogens or insects, which is important if the current forest cover is to be conserved (Ayres and Lombardero, 2000).
3.6 AGRICULTURE
Agriculture in the Atlantic region is a diverse, highly integrated sector representing 4% of the farms in Canada and utilizing 2% of Canada ’s agricultural land area (Statistics Canada, 2001a). In 1999, the value of the agri-food industry to the Atlantic economy ranged from just over 2% (NL) to over 12% (PE) of provincial GDP (Agriculture and Agri-Food Canada, 2005). Atlantic Canada produces 45% of Canada ’s potatoes, 39% of the country’s berries and grapes, and 4.3% of the milk (Statistics Canada, 2001a). Throughout the region, processing of agri-food products remains a significant part of economic activity (Hauer et al., 2002), resulting in more than 50% of the total economic activity in the sector (Krakar and Longtin, 2005).
Agriculture is highly dependent on climate. The growth of crops relies on a sufficiently long period of favourable temperature and timely rainfall. For animal production, climate controls when and if animals can be pastured, as well as the expenses associated with maintaining suitable animal shelter. For Atlantic Canada, the projected changes in climate present both opportunity and risk (Wall et al., 2004). Opportunities include growing higher value crops as a result of longer growing seasons, whereas risks are associated mainly with the increased frequency of extreme events, potential changes in pests and diseases, and uncertainty in global markets.
3.6.1 Sensitivities
An increase in climate variability and the frequency of extreme events would adversely impact the agricultural industry. A single extreme event (later frost, extended drought, excess rainfall during harvest period) can eliminate any benefits from improved ‘average’ conditions. Climate scenarios suggest increased climate variability, including increases in the frequency of hot days during the growing season, heat waves, number of cold days, late spring and early fall frosts, and numbers of consecutive dry days and intense precipitation events (Lines et al., 2003; Lines and Pancura, 2005), all of which have the potential to dramatically impact agricultural production. A warmer, wetter growing season may increase the potential for forage production, but could be offset by greater winter kill resulting from warmer winters (B élanger et al., 2001). Similarly, although warmer summer conditions may allow for a greater range of grapes and/or tree fruits, more extreme weather during the spring and fall could adversely affect the productivity of these crops. Fruit trees, which cannot be readily moved, are more susceptible to damage than are crops that can be replanted in subsequent seasons. The ability to respond to extreme events is related to the overall economic status of the sector (see Box 5) and the suitability of programs to deal with economic risk, such as income stabilization and crop insurance.
BOX 5 - Adaptive capacity in the Atlantic agriculture sector
There are several factors that affect the adaptive capacity of Atlantic Canada’s agricultural sector, including the current economic state of agriculture, demography of producers, health of rural communities and ability to deliver new technologies (Wall et al., 2004).
Atlantic Canada’s agricultural producers in 2003 were, on average, 53 years of age. A total of 43% had postsecondary education (the highest percentage in Canada) and 36% planned to retire by 2008 (Aubin et al., 2003). The majority of Atlantic agricultural operations are sole proprietorships (55%). Only 28% of operators plan to expand in the coming years (lowest in Canada; Aubin et al., 2003). The demographics of Atlantic agriculture represent both an opportunity and a threat in terms of response to climate change. The opportunity to recruit new, highly educated individuals into agriculture would enhance the capacity of the sector to adapt. The threat is that, because of the current economic climate, few young persons are electing to farm. This trend is not new: since 1981 there has been a 27% decrease in the number of farms in Atlantic Canada (Statistics Canada, 2001a), the highest rate of loss in Canada. This represents a significant vulnerability for agriculture in the Atlantic region.
There are also vulnerabilities associated with institutional support for agriculture, such as agricultural extension and breeding programs. Enrolments in faculties of agriculture have been in decline nationwide during the past 10 years. In response, many of the agriculture schools have become primarily environmental schools and fewer agricultural scientists are being trained. This diminished capacity will adversely affect the ability to identify and deliver the information needed to modify management practices to adapt to climate change, thus increasing sectoral vulnerability.
Extreme climate events can also increase agricultural impacts on the environment (Coote and Gregorich, 2000; De Kimpe, 2002). The primary environmental issues associated with agriculture in the Atlantic provinces are water quality, soil quality and, to a lesser extent, air quality. Atlantic producers acknowledge the importance of these impacts, identifying water pollution (52%) and soil erosion (47%) as the most significant environmental impacts of agriculture (Aubin et al., 2003). The past decade has seen efforts to implement management practices, such as improved crop rotations, soil conservation measures and more effective use of nutrients, that minimize environmental impacts. In many areas of Atlantic Canada, the management of manure is the primary environmental concern (e.g. Kings County, NS); in other areas, impacts are largely associated with intensified crop production (e.g. soil erosion and pesticide runoff from intensive potato production; Milburn et al., 1995).Water quality is of particular concern in Prince Edward Island, where groundwater is the only source of drinking water. Flooding increases the potential for agricultural impacts on surface water and groundwater.
An important limitation for agriculture in the region is the quantity and the agricultural capability of the soils. The soils are, for the most part, relatively fragile, and many have been degraded as a result of erosion, compaction and loss of organic matter related to a reliance on shorter, less diverse crop rotations and reductions in soil residue cover (Figure 26). The soils of Prince Edward Island and New Brunswick are most intensively managed, with 67% and 38% of the agricultural land, respectively, being cropped in 2001 (Statistics Canada, 2001a). Nova Scotia and Newfoundland have lower percentages of cropped land (29% and 21%, respectively) and have a greater predominance of permanent cover management systems, such as pasture and hay production. In Newfoundland, the amount of land available for arable production represents a significant constraint on the adaptation to climate change. Sustaining the productivity of agricultural soils and preventing increased impact on the surrounding environment will be important considerations in adapting to climate change (Coote and Gregorich, 2000).
The magnitude of relative changes in temperature and precipitation during the growing season (see section 2.1) will be important in determining adaptation in the agriculture sector. Bootsma et al. (2005a, b) examined the potential impact of changes projected by the first-generation coupled Canadian General Circulation Model (CGCM1; Boer et al., 2000) under emission scenario IS92a. Based on an increase in effective growing degree days and a decrease in growing-season water deficits (defined as the amount by which evapotranspiration exceeded precipitation) over much of the region for the 2040 to 2069 period, Bootsma et al. (2005 a, b) concluded that increased use of corn-soybean-cereal rotation, similar to that currently practised in southern Ontario, may give the best results. The result would be a doubling of income from these lands relative to their current use. In this analysis, the authors were not advocating the adoption of corn-soybean--cereal rotation but merely presenting an example of the potential implications of a change of climate on agricultural production. The study also emphasized that local climate will demand different adaptive responses across the region.
A warmer, wetter future would allow the growth of longer season, higher heat unit varieties and crop types. Given current market conditions, this would likely result in greater production of such cash crops as corn and soybeans, and the growth of more diverse tree fruits. Other market opportunities, such as the potential for producing feedstock for biofuel (e.g. ethanol and biodiesel) production or increased demand for organic foods, will influence the mix of crops being grown.
Climate change will also impact pest populations and their predators (Coakley et al., 1999). The warmer, wetter conditions predicted for Atlantic Canada will tend to favour a more diverse pest population (Rosenzweig et al., 2000). The complex interaction between crop pests, their predators and crop growth makes prediction of the potential impacts of climate change difficult. The rate of change in the size of pest populations and the spectrum of pests represents a key knowledge gap for agriculture. The time required to develop and adopt pest control approaches is significant, particularly if they require the registration of new chemical control agents (Coakley et al., 1999). The size of the Atlantic agricultural market is small, so the costs of developing and registering new products may not be considered economically viable. Furthermore, the development and dissemination of biological control agents or cultural practices used to control pests in organic production are difficult to achieve quickly.
As in crop production, the impacts of climate change on animal production are multifaceted, and include increased production costs resulting from increased energy demand to introduce artificial cooling of livestock buildings. In addition, animal diseases and their spread are significantly influenced by climatic conditions.
Bees have high economic value as pollinators in agriculture in Atlantic Canada. The apifauna is already under pressure from invasive species as well as parasitism and disease, and are likely to be negatively impacted by climate change (Richards and Kevan, 2002).
3.6.2 Adaptation
Adaptation to climate change for agriculture in Atlantic Canada will require a re-examination of cropping systems, including crop selection and soil management practices. There are many factors that influence the selection of crops, including agronomic, economic, environmental, social and cultural considerations. The limited analyses performed to date have generally focused on economic and, to a lesser degree, agronomic considerations. For example, Bootsma et al. (2005b) did not assess the range of cropping systems that might be suited to the projected future climate, whether the soils of the region could support the proposed change in cropping practices, the relationship to existing markets, or the suitability of current agronomic knowledge and infrastructure. All of these factors will influence future adaptation decisions.
It is also important to assess the potential impacts of proposed new cropping systems. Although a warmer and wetter future would allow for the adoption of more intensive cropping systems (Bootsma et al., 2005b), this transition would likely increase impacts on water as a result of increased soil erosion and leaching. The integration of animal and crop production is also an important consideration. One of the current issues in some areas is the concentration of animal production within a limited land-base. New cropping systems may provide an opportunity to address this issue.
The increasing demand for organically produced products and for the production of organic products within the region (Webb, 2002; Connell and Morton, 2003) could also shape future cropping practices. Although there are no climate impacts that are unique to organic production systems, the heavy reliance of these systems on legumes and the cultural control of pests may make management in a changing climate particularly challenging.
Perennial crops, including berry, grape and orchard industries, are economically important within the region. Warmer, wetter summers may expand the range of species and varieties that can be grown, but warmer winter temperatures may also result in increased winter damage to these crops (B élanger et al., 2001). Adaptation will also likely involve increased management of water on farms. These measures could include both improved drainage and erosion control, to facilitate the rapid removal of water from fields resulting from intense precipitation events, and on-farm or regional water management/storage to allow for irrigation during periods of drought. The restoration and maintenance of wetlands to process water from agricultural fields, by attenuating the flow of water to streams and retaining nutrients and sediments, is another important consideration.
3.7 TRANSPORTATION
Transportation in Atlantic Canada contributes significantly to Canadian, and especially provincial, GDP (Table 2) and to the Atlantic per capita economy (Table 3). Impacts on transportation will directly affect other sectors, such as manufacturing, tourism, urban growth, supplies and trade. In turn, changes in other sectors will influence demand for transportation (Yevdokimov, 2003). A warmer climate with varying precipitation changes will result in direct and indirect impacts on the transportation sector (Burkett, 2003). Transportation is linked to many socioeconomic activities (Zimmerman and Cusker, 2001; Transport Canada, 2003; Yevdokimov, 2003).
Province | Expenditure (millions of dollars) |
Percent of total Canadian GDP |
Percent of total provincial GDP |
---|---|---|---|
Newfoundland and Labrador | 448.7 | 1.1 | 3.5 |
Prince Edward Island | 74.4 | 0.2 | 2.4 |
Nova Scotia | 1 015.0 | 2.4 | 4.3 |
New Brunswick | 1 011.6 | 2.4 | 5.4 |
Province | Expen-diture (millions of dollars) |
Expen-diture per capita (dollars) |
Percent of total provincial personal expen-ditures |
Percent of total Canadian personal trans-portation expen-ditures |
Percent of provincial final domestic demand |
---|---|---|---|---|---|
Newfoundland and Labrador | 1 452 | 2 801 | 15.2 | 1.4 | 1.4 |
Prince Edward Island | 372 | 2 711 | 14.2 | 0.4 | 8.1 |
Nova Scotia | 2 720 | 2 711 | 14.2 | 2.6 | 8.2 |
New Brunswick | 2 240 | 2 982 | 15.8 | 2.2 | 9.1 |
Canada | 103 131 | 3 257 | 15.0 | 100 | 8.9 |
3.7.1 Sensitivities
Road
Road transportation is by far the largest component of the transportation sector in Atlantic Canada. Changes in climate that will affect road systems include periods of extreme heat and cold, increased freeze-thaw cycles and reduced ice cover. Greater numbers of hot days, as observed in interior New Brunswick and Nova Scotia, will likely lead to pavement softening (Mills and Andrey, 2003). Road damage resulting from both hot weather failure and enhanced frost heaving would leave regions susceptible to disruption of supplies and services.
For many coastal communities, sea-level rise will require coastal roads to be moved or rebuilt at higher elevations to avoid or reduce flooding. In some areas that are experiencing coastal erosion, roads have already been moved and will have to be moved again. For example, Highway 117 from Bouctouche to Saint- Édouard has been moved twice during the past three decades (Arsenault, pers. comm., 2004). In southern New Brunswick and northwestern Nova Scotia, Acadian dikes have reduced the impact of sea-level rise on roads by protecting land from erosion and flooding. However, these dikes were built more than a century ago and the maintenance they require may make them an expensive means of adaptation (Shaw et al., 1998). Storm damage to roads is also a concern (Figure 27).
Marine
In the Atlantic region, marine transportation is composed mainly of short-sea shipping (i.e. over short distances without crossing open oceans and within coastal waters). In addition, marine transportation includes fishing harbours, small craft harbours and ferry services (Fisheries and Oceans Canada, 2006). Recreational harbours are currently growing in significance throughout the region. Ferry services, provided by Marine Atlantic Inc., Bay Ferries and Northumberland Ferries, are an important economic component. In 2004, 419 548 passengers and 223 044 vehicles moved between Newfoundland and Labrador and Nova Scotia (Transport Canada, 2004). As well, international cruise ship traffic has been increasing in eastern Canada. Halifax saw 212 000 passengers in 2004 (Halifax Port Authority, 2006) and Saint John had 138 622 passengers the same year (Saint John Port Authority, 2005). Cruise ship visits are seen as economically important, and projects to deepen harbours to accommodate larger vessels have been undertaken in Charlottetown, PE and St. John ’s, NL.
Little work has been done to evaluate sensitivities of the marine transportation system, especially for waterways. Catto et al. (2006) investigated the effects of storm winds and surge activity on marine and road transportation through Channel-Port aux Basques, NL, and concluded that increasing easterly wind strengths posed a potential hazard. Weather-related delays to the Marine Atlantic ferry service resulted in an economic cost of more than $5 million in 2004 (Catto et al., 2006). Wharf construction will have to consider sea-level rise and storm events, and wharves should be raised to avoid inundation (Mills and Andrey, 2003). Although breakwaters are not always recommended during the installation of a new wharf, due to their potential to interfere with current patterns, increased storminess could lead to increased reliance on these structures to reduce impacts and infrastructure damage (McLean et al., 2001).
Many marine vessels currently in operation were built within the last 30 years and will therefore remain in operation for many more years. Although some larger vessels could cope with changes in marine weather conditions, there will be a need for retrofitting (Green et al., 2004).
Warmer winter temperatures and greater ice-free time could facilitate transportation in winter, spring and fall (Easterling, 2002; Langevin, 2003), enhancing shipping in some parts of the region. This phenomenon is already being observed in northern parts of the Atlantic provinces, such as the coast of Labrador, where marine shipping is continuing for a longer season. Gradually, as winter ice cover diminishes, marine shipping is likely to become increasingly important for the transportation of goods and services to northern regions (Goos and Wall, 1994).
Demand for emergency services provided by the Canadian Coast Guard will likely increase as a result of more frequent storms and extreme events (Burkett, 2003). Reduction in the length of the winter period and less sea ice, especially in areas such as the Northumberland Strait, could lead to different types of emergencies, including ice-shove activity. The ability to accurately identify the location of ice blocks is necessary to avoid an increase in the number of incidents. Real-time information and integrated management are important for all aspects of marine transportation and safety.
Rail
Very little research has been carried out on climate change impacts and adaptation related to rail transportation in Atlantic Canada. Railroads play an important role in New Brunswick and Nova Scotia. The main impact of concern is the potential disruption of the critical link through the Chignecto Isthmus (Tantramar Marshes) due to storm-surge flooding or destruction or damage of the Acadian dikes (Forbes et al., 1998; Shaw et al., 1998). Management plans could be continuously revised to adapt to change in sea level rise. Rail transportation in Maritime Canada is also exposed to changes in market and trade arenas (Nederveen et al., 2003). Its relative lack of flexibility for locations and schedules, as well as the need for transfer onto a truck at distribution stations, make it difficult to modify.
Air
Problems with ice and fog are the main climate impacts likely to occur at Atlantic Canada ’s airports. Changes in cloud cover and winter temperatures could influence the use of de-icing for aircraft. Flooding and storm activity could impact airport operations at Stephenville, NL. Airports in Halifax, NS, Saint John, NB and St. John ’s, NL may experience an increase in the number of delays or closures due to storms. Enhanced frost activity would shorten the lifespan of paved runways at airports. The social and economic consequences may be significant for communities that currently rely on aircraft, especially during the winter months, for everything from food supplies to access to medical services.
3.7.2 Adaptation
Transportation decisions and investments are generally made at the provincial level (with some national regulations and private investment). Identification of key facilities or locations that may be impacted by climate change, such as wharves, terminals and gas stations, is an important starting point (Potter, 2003). Assessing the resiliency of infrastructure to storms and extreme events, and identifying the emergency measures and resources needed to respond to extreme events is also recommended (Transport Canada, 2003).
With a greater frequency of freeze-thaw events in winter months, changes to road maintenance and salt usage will be required in order to reduce pavement damage and improve safety. In extreme cold or heat waves, roads tend to be affected by changes in temperature, leading to greater road damage and more dangerous driving conditions. Currently, few adaptations to such conditions are available. Road safety will continue to be a priority consideration.
Major transportation projects will have to consider climate change (Almusallam, 2001; Burkett, 2003). As vehicles and related infrastructure are short lived (most less than 25 years), changes could be implemented to facilitate cost-effective replacement using improved designs (Mills and Andrey, 2003). Bridges with a longer expected lifespan will have to be examined in light of continued sea-level rise and/or greater flooding in streams. New structures should be located outside impact areas associated with sea-level rise (Zimmerman and Cusker, 2001).
3.8 ENERGY
3.8.1 Sensitivities
Priority energy issues related to climate change adaptation for Atlantic Canada include changes in supply and demand, as well as impacts on exploration, production, transportation/transmission and other infrastructure. Relative to other sectors, little research has been conducted on the impacts of climate change on the energy sector in Atlantic Canada (Bell and McKenzie, 2004). Currently, load forecasts for electricity do not include climate change and are not calculated as far in advance as 2020 (H. Booker, Market Advisory Committee, NB, pers. comm., November 28, 2005). There is tremendous variability between the Atlantic provinces in terms of current energy mix for electricity production (Table 4). For example, in Newfoundland and Labrador 97% of electricity production (2002) was from hydro, whereas electricity in New Brunswick was produced from coal, oil, nuclear and hydro. Prince Edward Island purchased most of its electricity from New Brunswick and generated small amounts from wind turbines and oil, whereas Nova Scotia relied most heavily on coal and oil for electricity production (Table 4).
Province | Coal (%) | Oil (%) | Natural gas (%) | Nuclear (%) | Hydro (%) | Other (%) | Total Atlantic production (2002) |
---|---|---|---|---|---|---|---|
New Brunswick | 32 | 29 | 0 | 20 | 15 | 4 | 24.00 |
Newfoundland and Labrador | 0 | 3 | 0 | 0 | 97 | 0 | 59.62 |
Nova Scotia | 66 | 23 | 0 | 0 | 9 | 2 | 16.35 |
Prince Edward Island | 0 | 10 | 0 | 0 | 0 | 90 | 00.03 |
Total | 18 | 13 | 0 | 5 | 63 | 1 | 100.00 |
Electricity demand currently peaks in the winter in Atlantic Canada. Increased temperatures in winter will decrease energy demands for heating, whereas increased summer temperatures will result in increased electricity demands for air conditioning and refrigeration. This shift in demand would also result in a shift from direct utilization of fossil fuels for heating to electricity for cooling. Increasing demand for electricity from the northeastern United States (Natural Resources Canada, 2003a; Energy Information Administration, 2005) may result in pressure to increase energy exports.
Energy infrastructure is sensitive to impacts from extreme weather events, as evidenced by storms in 2004 that caused $12.6 million and $4 million in damage to electricity lines in Nova Scotia and New Brunswick, respectively (Bell and McKenzie, 2004). Weather events can produce generation and transmission inefficiencies, resulting in loss of power due to storms, such as occurred in Nova Scotia in 2006, New Brunswick in 2002 and Newfoundland in 1984 (Catto, in press).
Hydroelectricity is an important electricity production method in Newfoundland and Labrador. Long-term changes in seasonal and annual precipitation would affect overall generation capability, although electric power systems with dams and reservoirs will likely be able to adjust their operating practices to accommodate these changes (cf. St. George, 2006). More rainfall does not necessarily lead to more water in rivers and lakes, due to increased evapotranspiration resulting from higher temperatures. Reduced stream flow (Bruce et al., 2003) will likely have an impact on generation during dry summers, but higher levels of precipitation during the spring and autumn would help maintain reservoir levels, thus reducing the overall impact.
Wind generation (Figure 28) has become the fastest growing renewable energy supply in Atlantic Canada. Wind atlases show wind energy resources in Prince Edward Island (Gasset et al., in press) and Nova Scotia (Nova Scotia Wind Energy Project, 2004). Newfoundland has no wind farms at present, although the Newfoundland government has issued a call for proposals for a 25 MW facility (see Canadian Wind Energy Association, 2006a) and more than 10 areas throughout the province have been proposed for wind turbine development by private companies. There may be as much as a 10% reduction in summer winds in the Atlantic provinces by mid-century, although no change is anticipated for winter winds (Price et al., 2001). Ice storms can pose threats to wind turbines, requiring them to temporarily shut down to avoid damage (American Wind Energy Association, 2003). However, the impact of ice buildup on turbine blades appears limited (Australian Wind Energy Association, 2006; Canadian Wind Energy Association, 2006b).
Offshore petroleum exploration and production is sensitive to changes in storms, sea ice and icebergs. Reduced sea ice in the Atlantic region (Drinkwater et al., 1999; Hill and Clarke, 1999; Hill et al., 2002) may allow greater offshore activity, although high variability in sea-ice extent may make it difficult to predict and prepare for events, such as that which occurred near Sable Island in spring 2004, when sea ice forced the temporary abandonment of the Canadian Superior platform, at significant expense (Bell and McKenzie, 2004). Coastal energy infrastructure, such as generating plants (Point Lepreau and Coleson Cove, NB; Lingan/Aconi NS; Holyrood, NL), oil refineries (Saint John, NB; Dartmouth, NS; Come By Chance, NL), proposed liquefied natural gas terminals (Saint John, NB; Bear Head and Goldboro, NS), and where offshore pipelines come ashore (Goldboro, NS), may be susceptible to impacts from sea-level rise, storm surges and coastal erosion.
3.8.2 Adaptation
Adaptation to climate change by the energy sector in the Atlantic provinces will require re-examination of design standards for transmission and distribution infrastructure, to enable it to better withstand extreme weather events. Anticipation of changes in demand (both seasonally and by fuel source), contingency planning for extreme events and taking advantage of new and improving opportunities will also be important. Energy conservation, increasing grid reliability through diversification, and resource modelling of wind, solar and biomass energy are potential no-regrets adaptations.
3.9 TOURISM
3.9.1 Sensitivities
Tourism is currently the largest industry in Prince Edward Island (Government of Prince Edward Island, 2004) and is a major component in the economies of all four Atlantic provinces. Tourism is a vital component in the economic sustainability of most communities along the Atlantic coastline and is seen as a key component in the economic revitalization of formerly fishery-dependent communities. Recent increases in summer economic activity in some rural communities are notable.
Environmental conditions are an important factor in decisions made by prospective tourists, particularly where the natural environment is among the destination’s primary attractions (Braun et al., 1999; Scott and Suffling, 2000). As climate change occurs in Atlantic Canada, tourism may be affected both positively and negatively (DeBaie et al., 2006). The impacts of climate change on tourism, and the impact of tourists on the environment, must both be considered.
Rising sea level, increased coastal erosion, beach narrowing and coarsening, and increased storm activity all have negative impacts on tourism in coastal regions (Mimura, 1999; Uyarra et al., 2005). Damage to infrastructure represents an additional concern. Coastal development and the construction of protective infrastructure would restrict the movement of the beaches and sand dune systems landward. Wave energy would be focused in progressively smaller areas, resulting in the preferential removal of sand and causing beaches to become narrower and coarser. Where coupled with restrictions on sand supply, the result would be to produce gravel beaches, which are less attractive for most tourists. This would have important economic impacts on the communities that rely on tourism for sustainability (Cambers, 1999; Fish et al., 2005; Uyarra et al., 2005).
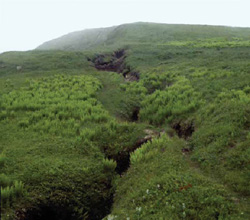
FIGURE 29b: Excessive tourist foot traffic facilitates erosion at Cape St. Mary's Ecological Reserve, NL.
Geomorphic stresses induced by climate change, in combination with increased visitation, lengthening of the tourist season and enhanced tourist use of coastal areas, have resulted in accelerated erosion of coastal tourist sites throughout the region and could have an impact on the long-term sustainability of coastal tourism (Daigle et al., 2006). Increased foot traffic and vehicular access to beaches and backing dunes (Figure 29) have also had an impact (e.g. Catto, 2002, 2006a, b; Catto et al., 2002). National and provincial parks are also vulnerable to sea-level rise and storm surges.
Iceberg viewing has become a popular form of tourism along the Newfoundland coastline. From the mid-1990s to 2003, iceberg numbers increased due to glacial calving in Greenland (Petersen, 2005), posing difficulties for petroleum-related operations but providing opportunities for tourism. During the period 2004 to 2006, iceberg numbers and sightings decreased along the northeastern Newfoundland coastline, causing concern for local tourism-related businesses (e.g. G. Noordhof, L ’Anse-aux-Meadows, pers. comm., 2006). The number of icebergs is directly related to glacial activity in Greenland and water temperatures in the Labrador Sea (cf. Canadian Institute for Climate Studies, 1999 –2005; Hadley Centre, 2006).
In the winter, changes in the duration and distribution of snow will impact winter recreational activities, such as snowmobiling and cross-country skiing (Abegg et al., 1998; Harrison et al., 2001; Scott et al., 2003). Climate change would reduce the number of days suitable for snowmobiling by 38 to 62% by the 2020s in eastern North America, compared to conditions in the 1970s (McBoyle et al., 2006). By 2050, the predicted snowmobiling season would be less than one week in Sydney and Gander, and between 0 and 20 days at Fredericton (McBoyle et al., 2006). Snowmobile sales declined by 38.4% in Canada and the United States between 1997 and 2005, while ATV sales increased by a comparable percentage. Manufacturers have adapted by increasing ATV production, and prospective riders are purchasing ATVs as all-terrain, all-weather vehicles.
3.9.2 Adaptation
In Atlantic Canada, tourism marketing is increasingly geared towards longer seasons, varied activities and diverse demographic audiences. Visitors are seeking cultural, historical and recreational opportunities. Tourist preferences are subject to fashion, transportation costs, exchange rates and perceived security issues, such that a single event may have disproportional, unforeseen and persistent effects.
Tourist operators and promoters, including multiple levels of government, can change advertising campaigns in response to changed conditions. Some aspects of tourism in Atlantic Canada, including longer summer seasons, are favoured by ongoing climate change. As warming increases more rapidly in central North America and western Europe, the relatively cool summers of Atlantic Canada are increasingly seen by tourists as an attractive environment for vacationing or a summer residence.
Comprehensive assessment of the stresses induced by tourist activity at coastal sites is needed to recognize the potential for erosion and changes in beach sediment before substantial degradation occurs. Important tourist sites, such as Panmure Island Provincial Park, PE and the dune at Bouctouche, NB, are currently at risk from coastal erosion, and protection work is needed to care for such sites. Tourism may be addressed in the design of coastal protection. For example, aesthetic concerns can influence the design of shoreline protection (as in Victoria Park, Charlottetown, PE), and structures may be designed to simultaneously provide recreational walking trails (as in Summerside, PE and Trout River, NL).
At Prince Edward Island National Park, many repairs have been needed as a result of storm surges. The winter storm of 2004, which occurred when no ice cover was present, was particularly damaging to park infrastructure. Retreat has been used as an adaptation approach for some campground shelters. Armour stone has been used in some road sections and to protect bridges. Numerous repairs to roads, boardwalk and other infrastructure have also been required. In Kouchibouguac National Park, some structures have been solidified, elevated, moved or removed seasonally in reaction to, or to prevent damage from, storms. These modifications have been ongoing since the park's creation.
3.10 COMMUNITIES
3.10.1 Sensitivities
Impacts from sea-level rise, modifications to wave regimes, storm surges, changes in the severity and frequency of storms, and alteration in the duration of ice cover will all affect communities in Atlantic Canada (McLean et al., 2001; Thompson et al., 2005; Catto et al., 2006). Recent flooding and hurricane events, which have resulted in property and infrastructure damage, injury and death, isolation from emergency services and power outages, demonstrate the vulnerability of Atlantic communities to climate impacts.
The geographic setting of communities (inland versus coastal) in the Atlantic region will result in different types of impacts with varying levels of both sensitivity and vulnerability. Coastal communities, such as Annapolis Royal and Halifax, must deal with impacts of storm surges (see Boxes 6 and 7). In contrast, inland communities are more susceptible to changes in temperature and precipitation, which impact water supplies, flooding, agriculture and forests. A distinction is also apparent between rural and urban communities, although differences in this case are more related to the ability to adapt. For all communities, climate influences are superimposed on other political, socioeconomic and technological factors that influence vulnerability (see Boxes 4, 5).
Rural communities in Atlantic Canada are, in many instances, facing economic difficulties resulting from their dependence on a single natural resource. Climate change, by placing additional pressure on natural resources such as marine species or agricultural crops, could worsen the situation for many communities. In this context, climate change should not be seen as an independent, solely dominant factor, but as one of several stressors acting on fishing, forestry and agricultural communities. The interaction of numerous stress factors substantially increases the vulnerability of rural, single-resource-dependent communities throughout Atlantic Canada.
Health and social effects will also be associated with the direct impacts of climate change on sectors such as tourism, agriculture and fisheries (Brklacich et al., 2007). Loss of income or employment can produce stress-related disorders and mental illnesses (Sowder, 1985; Health Canada, 2005). Severe weather events and associated natural hazards can result in people being dislocated and forced to temporarily reside in crowded shelters, which increases the risk of disease outbreak. Residents are also affected by the stress induced by such events, leading to a variety of mental health impacts, including depression from financial loss, injuries and relocation (Abrahams et al., 1976; Noji, 1997; Greenough et al., 2001; Soskolne and Broemling, 2002; Soskolne, 2004). Psychological effects commonly persist for several years following a disaster (Bennet, 1970; Powell and Penick, 1983; Sowder, 1985).
For interior communities, there are health risks associated with contamination of groundwater and surface water caused by heavy precipitation, as surface runoff may pollute water supplies with animal wastes and pesticides. Extreme precipitation events may also overwhelm water treatment facilities or cause sewers to overflow, resulting in further contamination (Health Canada, 2005; New Brunswick Department of Health, 2005). Increases in heavy precipitation events will also affect storm-water systems. Sensitivity depends on the nature of the infrastructure and the intensity of precipitation events (Watt et al., 2003). Inquiries within some urban communities in New Brunswick revealed that present storm-water management does not take projected increases into account, although some communities do incorporate a certain level of uncertainty (e.g. 20% in Moncton, NB).
BOX 6 - Annapolis Royal: coastal flood and storm-surge mapping
The Tidal Surge Study by the Clean Annapolis River Project (CARP), a citizen-based group, is an exemplary case of a project that increased the adaptive capacity of a community in the face of climate change (Belbin and Clyburn, 1998).
The town of Annapolis Royal, located at the west end of the Annapolis Valley on the northwest coast of Nova Scotia, was concerned about increased risk from flooding during perigean spring tides and extreme weather events. Annapolis Royal is in danger of flooding because much of the region is below sea level, reclaimed in the seventeenth century by the Acadian settlers using dykes. Additionally, the land has sunk lower over time. Roads, bridges and buildings have already been flooded, putting the town at risk.
All the data collected for this project were obtained from existing sources. Historical records regarding past extreme events were collected from museums, newspapers and historical societies within the region. The Saxby Gale of October 4–5, 1869 was used as model for sea-level predictions.
A few centimetres can be the difference between a disastrous flood and a non-event. Maps with 2 m contours and 0.1 m spot elevations were used to determine the locations at greatest risk from tidal-surge flows, and the regions most in danger of flooding. The maps revealed particular concerns, including the fact that the fire department is situated on a small rise (Figure 30). During flooding, it would be on an island, isolated from the community. Following the study, the emergency rescue equipment that was previously stored solely at the fire department was relocated around the town. The fire department also acquired a boat to be used for transporting personnel and equipment, and to provide access to the mainland during a flood.
The Emergency Measures Organization (EMO) has begun to closely monitor the patterns and heights of the tides in the region. Monitoring has now become a province-wide action, as it is recognized that the danger is not localized in the Annapolis region.
Another important action taken as a result of this project was the enactment of a mock disaster scenario. Annapolis Royal acted out step-by-step procedures of what would occur in an emergency. This allowed the town to become aware of what steps needed to be taken to prevent more damage or potential harm to people in a real disaster. Using a paper simulation, part of the town was evacuated and a county-wide scenario was built to deal with the implications of a storm surge. New mechanisms have been established within EMO to allow a seamless melding of services. The results of the Annapolis Royal case study are currently being used to develop a toolkit for land-use planners (Parks, 2006).
This case study shows that small communities can take steps to prepare for the impacts of climate change. By determining the damages that could occur during an extreme weather event, the town was able to take preventive measures that reduce the risk of large-scale economic loss from flooding.
Atlantic Canada is recognized as one of four areas in Canada where air pollution is greatest, largely because of air masses from the eastern United States (Labelle, 1998). Ozone is the most common air pollutant; the national concentration of ground-level ozone increased by 16% between 1990 and 2003 (Statistics Canada, 2005c). An increase in heat waves, combined with air pollution, would increase the frequency of smog days in urban areas and associated health problems, such as asthma and other pulmonary illnesses, as well as heat stress and related illnesses (McMichael et al., 2003; Epstein and Rogers, 2004; Health Canada, 2005). Impacts of heat waves, smog events and airborne particulates from forest fires may be compounded as a result of climate change. Related illnesses and deaths, especially in urban areas, can be expected to rise as poor air quality aggravates cardiovascular and respiratory diseases (McMichael et al., 2003; Health Canada, 2005).
A total of 12% of Canadians under age 12, and 8% over age 12 have been diagnosed with asthma (Statistics Canada, 2005c), and the number of Canadian adults suffering from asthma increased from 2.3% in 1979 to 6.1% in 1994 (Health Canada, 1998). Atlantic Canada has some of the highest rates of asthma in the country (Public Health Agency of Canada, 1998; Canadian Council on Social Development, 2006). Heat waves and smog events can also increase the risk of strokes, with some studies showing a link between stroke and respiratory illnesses and environmental change (Epstein and Rogers, 2004). For example, increases in levels of suspended particulate matter (air pollutants) are positively correlated with increases in rates of myocardial infarction and pulmonary infection cases requiring hospitalization or resulting in death (Dominici et al., 2006; Murakami and Ono, 2006). As well, some authors underline the possible increase in allergens related to increases in CO 2, which will also affect respiratory health (Epstein and Rogers, 2004).
3.10.2 Adaptation
Although there has been much discussion on the capacity of different communities to respond to environmental changes (Pelling and High, 2005), there has been less research focused on building community adaptive capacity (Smit and Pilifosova, 2003; Brklacich et al., 2007). Nevertheless, individuals, groups and municipal governments in Atlantic Canada are already involved in adaptation efforts, mostly in the form of coastal protection.
Rural and urban communities in Atlantic Canada differ widely in their demographics, economic resiliency and robustness. Consequently, their ability to adapt, and therefore their vulnerability, differ substantially. Urban centres generally have greater financial, institutional and human resources to meet challenges, including climate change. Adaptations being undertaken by the Halifax Regional Municipality are currently beyond the means of rural communities.
Local governments are actively involved in adaptation efforts. Following severe damage due to storm events, droughts and non-native insect invasion, the Halifax Regional Municipality initiated sustainable planning, which includes planning for climate change adaptation. The Climate SMART program works with the private sector to include greenhouse gas reduction and climate change adaptation measures in the decision-making process. The building of a wastewater treatment facility with provision for 3 m rise in sea level, and a thermal energy co-generation plant for hospitals and universities, are two initiatives that have emerged from Climate SMART (see Box 7). Another example of adaptation at a municipal level is from the town of Rexton, NB. This community is affected by tides and storm surges on the Richibucto River, which have caused erosion and flooding. Rexton has been actively protecting historically and culturally significant parts of the shoreline along the river for several decades.
BOX 7 - Reducing vulnerability through the Climate SMART Initiative
Halifax Regional Municipality (HRM) covers more than 5000 km2 and has a population of more than 350 000. It offers an international sea terminal and airport, as well as commercial, educational, research and technological centres for the region. In recent years, Halifax has experienced a number of extreme climate events, with increased impacts, damages and costs. The category 2 Hurricane Juan (September 2003) made landfall outside of Halifax and tracked across central Nova Scotia, causing extensive damage to property, infrastructure and the environment. Only a few months later, in February 2004, the severe winter blizzard that became known as ‘White Juan’, dumped almost 90 cm of snow on HRM in one day. The storm resulted in $5 million of unbudgeted snow removal costs and repairs to utility infrastructure. Such events have cost HRM, and its businesses and citizens, millions of dollars, the loss of several lives, disruption of services and great inconvenience. These events have also drawn attention to, and triggered an increased level of concern regarding, the potential impacts of climate change. Prior to Climate SMART, HRM did not have climate change planning strategies in place. Recognizing the increased risk to infrastructure, property and its citizens that could result from climate change, particularly projected increases in the frequency and/or intensity of extreme climate events, HRM looked for a mechanism to plan and implement climate change strategies. Climate SMART (Sustainable Mitigation and Adaptation Risk Toolkit) has been developed to help mainstream climate change mitigation and adaptation into municipal planning and decisionmaking. It is a collaborative partnership between the public and private sectors. Partners in the HRM prototype project include the Federation of Canadian Municipalities, Natural Resources Canada, Environment Canada, Nova Scotia Department of Energy, Nova Scotia Department of Environment and Labour, Nova Scotia Environmental Industries Association, members of ClimAdapt, several community groups and local businesses, and HRM. Climate Smart is the first initiative in Canada to advocate a fully integrated approach to addressing climate change at the municipal level. The HRM and its partners officially launched Climate SMART in March 2004. Principal tasks of the prototype HRM Climate SMART project include developing:
- vulnerability assessments and sustainability analyses;
- cost-benefit assessments;
- emissions management and mitigation tools;
- a plan for managing climate change risk;
- an emissions management and adaptation methodology, which includes methodologies for each sector of the community; and
- communications and outreach initiatives.
Several project components are already contributing to Halifax’s overall environmental strategic planning efforts. Future plans include defining and conducting risk and vulnerability assessments, and developing the adaptation management tools that will enable HRM to incorporate climate change into municipal planning and decision-making.
Many communities are requesting assistance from provincial governments for protection of their coasts, and all provinces are beginning to address the impacts associated with climate change. The Prince Edward Island Special Committee on Climate Change presented its final report in April 2005, recommending measures to protect coastal areas (Special Committee on Climate Change, 2005). The elimination of quarry permits for beaches and a revision of emergency response measures were also included in the report. The government of Nova Scotia released an Issues Paper in 2005 entitled Adapting to a Changing Climate in Nova Scotia: Vulnerability Assessment and Adaptation Options (Government of Nova Scotia, 2005). Nova Scotia has also worked on identifying the sensitivity of different coastal areas (Nova Scotia Department of Energy, 2001), although the report contains little in terms of adaptation measures. In Newfoundland and Labrador, an action plan containing measures to reduce greenhouse gas emissions, as well as some adaptation measures, was released in June 2005 (Government of Newfoundland and Labrador, 2005).
Many discussions with groups in New Brunswick have focused on the issue of governance, especially in rural areas. Rural areas of the province are grouped into Local Service Districts (LSDs), and a local committee of non-elected representatives makes recommendations to the provincial government regarding their needs. Many individuals think that this mechanism provides very little power to communities, and that demands regarding adaptation in their area are diluted among requests made by other LSDs (Martin and Chouinard, 2005). In the absence of other mechanisms, residents of the community of Pointe-du-Chêne took it upon themselves to take action following recent storm-surge events. A special committee organized an emergency shelter and is involved in discussions with different levels of government regarding investigation of long-term solutions to the flooding associated with storm surges.
The period of increased hurricane activity currently ongoing in the North Atlantic suggests a need for enhanced emergency preparedness and adaptation (Goldenberg et al., 2001). As a preventive measure, the Emergency Measures Organization has adopted a new awareness campaign to help citizens of Prince Edward Island better prepare themselves in case of hurricanes. The Canadian Hurricane Centre has also increased efforts to remind the population of the severity of hurricane warnings in order to improve preparedness (Environment Canada, 2004c).
One of the main obstacles to implementing adaptation remains the generally short-term view of developers and government officials relative to the long-term effects of climate change (Federation of Canadian Municipalities, 2002). The lack of resources is also a major obstacle that individuals and communities are facing in implementing adaptive measures (Federation of Canadian Municipalities, 2002). Protection structures are very costly, and conflicts between residents have occasionally resulted from the inability of some to contribute to structures deemed necessary by others (structures being more efficient if they are continuous along a given stretch of coastline).
There is a need for improved knowledge about climate change at all levels of decision-making. This will improve the adaptive capacity of communities, contributing to reduction of their vulnerability to climate change. Increasingly, this is being achieved by linking communities to research projects in ways that allow them to be consulted and to obtain an understanding of the potential impacts of climate change (e.g. Vasseur et al., 2006). One of the most effective means of accomplishing this is through the integration of climate change impacts and adaptive considerations into the Environmental Impact Assessment process.
Page details
- Date modified: