Key Concepts - CCR
Canadians are increasingly aware that climate change represents a fundamental challenge to our health and well-being, environment and economy. The vast majority of discussion at the public-policy level has focused on mitigation, the critically important response of reducing greenhouse gas emissions. Mitigation is essential to slow the rate, and ultimately to limit the magnitude, of climate change. There is, however, less awareness of the fact that, regardless of the success of global mitigation initiatives, further climate change and associated impacts are unavoidable (e.g. Intergovernmental Panel on Climate Change, 2007a). Even if greenhouse gas concentrations were stabilized, warming and sea-level rise would continue for centuries due to the nature of the climate system and feedbacks (Meehl et al., 2006; Intergovernmental Panel on Climate Change, 2007a). This assessment focuses on the need for adaptation in recognition of the reality that Canada's present climate is different from that of the recent past and will continue to change in future.
Adaptation actions undertaken by Canadian governments, industry, communities and individuals are, and will continue to be, based on implicit or explicit understanding of vulnerability. With respect to climate change, this involves considering how climate is likely to change, the probable impacts of these changes and the potential for adaptation. To understand vulnerability, authors of this assessment have drawn from a wide range of disciplines, ranging from the physical, biological and social sciences to economic analysis, and have integrated this information with other sources of knowledge, including local knowledge. Several key concepts that use terminology specific to the field, and convey explicit meanings that extend beyond the basic dictionary definitions, underlie this analysis. Rather than repeating explanations of these key concepts throughout the report, they are discussed in detail here. Note that a more extensive list of key terms in the field of impacts and adaptation is contained in the glossary to this report. For the remainder of Section 2 only, the first occurrence of words in the glossary is in bold italics.
2.1 ADAPTATION
Adaptation refers to any activity that reduces the negative impacts of climate change and/or positions us to take advantage of new opportunities that may be presented. Adaptation is needed to address the challenges of climate change, and represents a necessary complement to mitigation (reduction of greenhouse gas emissions; Box 1). Both the United Nations Framework Convention on Climate Change (UNFCCC) and the Kyoto Protocol include requirements for Parties (i.e. countries) to address adaptation. The goals of adaptation may include 1) alleviating current impacts (Füssel and Klein, 2006); 2) reducing sensitivity and exposure to climate-related hazards; and 3) increasing resiliency to climatic and non-climatic stressors (i.e. enhancing adaptive capacity). Successful adaptation does not mean that negative impacts will not occur, only that they will be less severe than would be experienced had no adaptation occurred.
There are many different types of adaptation (Table 2). Adaptation includes activities that are taken before impacts are observed (anticipatory) and after impacts have been felt (reactive). Both anticipatory and reactive adaptation can be planned (i.e. the result of deliberate policy decisions), while reactive adaptation can also occur spontaneously (i.e. autonomous, without planning). Planned adaptation is an iterative process involving four basic steps: information development and awareness-raising; planning and design; implementation; and monitoring and evaluation (Figure 1; Klein et al., 1999). In most circumstances, anticipatory planned adaptations will incur lower long-term costs and be more effective than reactive adaptations. Nevertheless, there are risks involved in implementing adaptation options to deal with an uncertain future, including opportunity costs (the use of resources that could otherwise be used for competing priorities) and the potential for maladaptation (see Mendelsohn, 2006).
BOX 1: Adaptation and mitigation
There are two categories of response to climate change: mitigation and adaptation. In the climate change literature, these two terms have clear and distinct definitions, and there are fundamental differences between them (see Table 1). Mitigation refers to "anthropogenic interventions to reduce the sources or enhance the sinks of greenhouse gases" (Intergovernmental Panel on Climate Change, 2001a). The goal of mitigation is to reduce or prevent changes in the climate system and, as such, mitigation focuses on the sources of climate change (Schipper, 2006).
Characteristics | Adaptation to Climate Change | Mitigation of climate change |
---|---|---|
Benefited systems | Selected systems | All systems |
Scale of effect | Local to regional | Global |
Lifetime | Years to centuries | Centuries |
Effectiveness | Generally less certain | Certain |
Ancilliary Benefits | Mostly | Sometimes |
Monitoring | More difficult | Relatively easy |
Adaptation, on the other hand, is concerned with addressing the consequences of climate change (Schipper, 2006). Adaptation refers to activities aimed at reducing or preventing the impacts of climate change on human and natural systems.
Although the two terms are distinct, adaptation and mitigation are also codependent. Mitigation, through moderating both the rate and magnitude of changes in the climate system, affects both the demand for, and the potential success of, adaptation options. Greater magnitudes of change will require more extensive adaptation, and greater rates of change make adaptation more challenging. In addition, there are some activities that can be considered both mitigative and adaptive. For example, planting trees in urban areas both increases greenhouse gas sinks (mitigation) and acts to cool surrounding areas (adaptation to increased temperatures). This codependency between adaptation and mitigation indicates the need for climate change policies that address the two responses simultaneously (Mendelsohn, 2006).
While the distinction between adaptation and mitigation is well established in the climate change community, not all disciplines use these terms in this way. The natural hazards community, for example, has long used the term 'mitigation' to refer to activities that reduce the impacts of natural hazards. For example, land-use planning that limits development in floodplains would be considered a mitigation measure in the natural hazards community but an adaptation measure in the context of climate change.
Many different groups, including individuals, organizations, industry and all orders of government, are involved in facilitating adaptation and in the choice and implementation of specific adaptation measures. Such measures are highly diverse, and may involve behavioural changes, operational modifications, technological interventions, and revised planning and investment practices, regulations and legislation. The role of governments includes the provision of information and tools, and the establishment of policy frameworks, that promote adaptation action (Stern, 2006).
Many climate change impact studies provide lists of potential adaptations. Such lists help exemplify the diverse range of adaptation responses, and many examples of these are presented in the regional chapters of this assessment. Nevertheless, they represent only a starting point in analysis. Decisions regarding the most appropriate adaptation response to address a specific impact, or suite of impacts, require understanding of the process of adaptation and the related concepts of vulnerability, adaptive capacity and resilience (see Sections 2.2-2.4). Adaptation will not take place in response to climate change alone, but in consideration of a range of factors with the potential for both synergies and conflicts. Attention must be paid to the feasibility, likelihood and mechanisms for adaptation uptake. Critical questions include the following (Smit and Wandel, 2006): "What can be done practically?", "Who will do it?" and "How will it be implemented?" Research on such questions is currently sparse in the field of climate change (Smit and Wandel, 2006).
2.2 VULNERABILITY
In the climate change literature, vulnerability refers to the degree to which a system is susceptible to, and unable to cope with, the adverse effects of climate change (Intergovernmental Panel on Climate Change, 2001a). The Intergovernmental Panel on Climate Change (2001a) states that "vulnerability is a function of the character, magnitude, and rate of climate variation to which a system is exposed, its sensitivity, and its adaptive capacity." As such, vulnerability integrates an external dimension, namely exposure to climate, as well as characteristics internal to the system under study (sensitivity and adaptive capacity; F üssel and Klein, 2006). It also necessitates an understanding of both biophysical and socioeconomic processes (Adger, 2006).
ADAPTATION | |||
Based on | Type of adaptation | ||
Intent | Spontaneous | nPlaned | |
Timing (relative to climate impact) |
Reactive | Concurrent | Anticipatory |
Temporal scope | Short term | Long term | |
Spatial scope | Localized | Widespread |
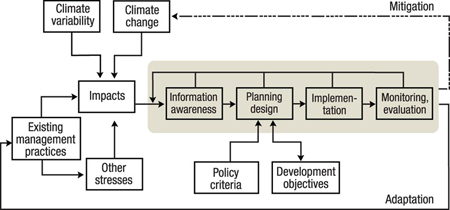
FIGURE 1: Conceptual framework showing (in the shaded area) the steps involved in planned adaptation to climate variability and change (from Klein et al., 2006).
Text version
Flowchart demonstrating the conceptual framework of the steps involved in planned adaptation to the impacts of climate variability and change which flow from information awareness, planning design (policy criteria and development objectives), implementation and monitoring and evaluation. These steps can either lead to mitigation, and thus climate change or to adaptation which includes changes to existing management practices, affecting other stresses and impacts of Climate change and variability.
As an example, the vulnerability of an agricultural operation to climate change requires understanding of how climate is likely to change (e.g. increased temperatures, more frequent droughts), the sensitivity of the system to that change (e.g. the relationship between crop yield and temperature and/or drought) and the potential for the system to adjust to the change (e.g. planting different crops, irrigation). Although this operation may be highly sensitive to climate change, in that crop yield is strongly controlled by temperature and drought, the system would not be considered highly vulnerable if effective adaptation measures, such as switching to more drought-resistant crops, are easy to implement.
The above example illustrates three other important aspects of vulnerability. First, by definition, vulnerability focuses on negative impacts - the "adverse effects of climate change" (Intergovernmental Panel on Climate Change, 2001a). It is well accepted, however, that climate change will bring benefits as well as negative impacts. In the example provided, increased temperatures may well lead to increased crop yields. Hence, adjusting activities so as to best capitalize on these benefits is also a recognized goal of adaptation. Second, the aspects of climate change most important for informing adaptation decision-making are rarely captured well in terms of the most commonly discussed climate parameters: changes in mean temperature and precipitation. In this example, more important considerations for crop yields may include the timing of precipitation, occurrence of extreme rainfall, growing degree-days and drought severity. Third, and most important, even if the vulnerability of a system is considered relatively low due to a high capacity to adapt, it may still incur significant impacts if adaptation actions are not implemented. In the example provided, if the operator continued to plant the same crop and made no other adjustments in the operation, they could experience severe negative impacts or would fail to benefit from new opportunities.
Recognition of the need to consider the ability of systems to adapt is what distinguishes vulnerability from sensitivity. Sensitivity does not account for the moderating effect of adaptation, whereas vulnerability can be viewed as the impacts that remain after adaptation has been taken into account. Although many early climate change impact studies focused primarily on sensitivity, it is now accepted that adaptation will strongly influence the magnitude of climate change impacts. Indeed, researchers have noted that "it is meaningless to study the consequences of climate change, without considering the ranges of adaptive responses " (Adger and Kelly, 1999). Most of the more recent climate change impact studies focus on assessing vulnerability, rather than sensitivity.
Assessing vulnerability requires consideration of the main stressors, both climatic and non-climatic, on a system or region, as well as the socioeconomic influences on adaptive capacity (see Section 2.3; F üssel and Klein, 2006). It is widely recognized that engagement of stakeholders represents a critical first step in assessing vulnerability (Lim et al., 2005). While impacts are frequently expressed quantitatively (e.g. percentage increase in productivity, dollar loss in revenue), vulnerability studies focus more on understanding the processes involved and influencing factors. The social and biophysical influences on vulnerability change readily over time and space (Adger, 2006). As a result, vulnerability is generally characterized, rather than measured, although advances in quantifying the concept are ongoing (see Adger, 2006).
2.3 ADAPTIVE CAPACITY
In the context of climate change, adaptive capacity is defined as the "potential, capability or ability of a system to adapt to climate change stimuli or their effects or impacts " (Intergovernmental Panel on Climate Change, 2001a). A system is a broad term, which encompasses all scales and types of units, including regions, communities, economic sectors, institutions and private businesses.
Adaptive capacity is a relatively new term in climate change research, first appearing in the scientific literature in about 1999 and not being cited frequently until 2003. The uptake and use of the term was likely spurred by the publication of the Third Assessment Report of the Intergovernmental Panel on Climate Change (2001), in which Chapter 18 ('Adaptation in the Context of Sustainable Development and Equity'; Smit et al., 2001) discussed the concept in detail. Adaptation and adaptive capacity are closely linked (Box 2), and enhancing adaptive capacity is a 'no-regrets' adaptation option that brings benefits regardless of the changes in climate. As such, adaptation approaches that focus on enhancing adaptive capacity are an effective way of taking action, despite the uncertainties inherent in projections of future climate (Smit and Pilifosova, 2003). By increasing adaptive capacity, vulnerability to current climate, future climate and oftentimes other stressors are reduced.
BOX 2: Contrasting adaptation and adaptive capacity
Adaptive capacity and adaptation, although related, are distinct terms in the climate change literature. Adaptive capacity is an attribute of a system, which provides an indication of its ability to adapt effectively to change. A system with a high adaptive capacity would be able to cope with, and perhaps even benefit from, changes in the climate, whereas a system with a low adaptive capacity would be more likely to suffer from the same change. Adaptation, on the other hand, refers to a process and/or specific action.
Building adaptive capacity is a component of adaptation strategies (Brooks et al., 2005), and a system with many adaptation options generally has a higher adaptive capacity than a system with few or none (Yohe and Tol, 2002). Some suggest that adaptive capacity can be viewed as the potential for adaptation and, when adaptive capacity is used to adapt, vulnerability is reduced (Brooks, 2003).
To address adaptive capacity, two key questions must be considered: "Adaptive capacity of what?" and "Adaptive capacity to what?" (Smit et al.,1999). One may, for example, consider the adaptive capacity of a farm (system) to increased aridity (climate change), or the adaptive capacity of a community (system) to more frequent heat waves (climate change). Adaptive capacity is influenced by a number of location-specific determinants, which depend upon the social, economic and institutional state of the system or region being studied (Figure 2). These determinants act to either constrain or enhance ability to adapt (Kelly and Adger, 2000), and vary in both space and time (Smit et al., 2001).
Past experience clearly influences adaptive capacity. Canada's highly variable climate contributes positively to the capacity of Canadians to adapt to climate change. Single events can impact adaptive capacity both positively and negatively (Smit et al., 2001).
For example, lessons learned from a recent storm surge should lead to improved preparedness for future storms, thereby enhancing adaptive capacity. However, if recovery from that same event exhausted financial resources available to assist flood victims, adaptive capacity could be diminished until those resources are replenished. Past events also influence perception of risk at the individual and institutional levels, which in turn affects the likelihood of proactive adaptation (Grothmann and Patt, 2005).
Adaptive capacity is difficult to measure. Proxy indicators, such as per capita income, education level and population density, have been used for some of the determinants (Yohe and Tol, 2002), but others are more difficult to assess. In addition, although adaptive capacity is most meaningful as a local characteristic, data availability frequently means that it can only be assessed at the national or regional level (Yohe and Tol, 2002).
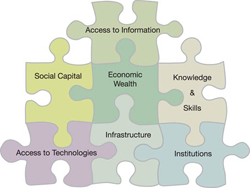
FIGURE 2: Determinants of adaptive capacity (adapted from Smith et al., 2003).
Text version
Determinants of adaptive capacity represented by puzzle pieces. Headed by access to information, followed by social capital, economic wealth, knowledge and skills, with the last later consisting of access to technologies, infrastructure and institutions.
For this assessment, authors focused on characterizing the factors that influence adaptive capacity within their region, in some instances extending this characterization to the system level (subregions or sectors). Although discussion of adaptive capacity at the local level is rare in the climate change literature, there is considerable potential to learn lessons from analyses of other disciplines, including emergency preparedness, economic development/diversification and food security. While generally beyond the scope of this assessment, such analysis represents a profitable direction for future impacts and adaptation research (see Chapter 10).
2.4 RESILIENCE
Resilience is defined as the "amount of change a system can undergo without changing state" (Intergovernmental Panel on Climate Change, 2001a). The term 'resilience' is not as commonly used in the climate change literature as 'adaptive capacity' or 'vulnerability'. Studies that do use the term tend to focus more on natural systems, rather than human systems, likely due to the term's roots in the field of ecology. Some researchers have modified the terminology for specific use in climate change studies, and now refer to 'eco-social resilience' or 'social-ecological resilience' (e.g. Adger, 2006).
As noted above, much of the terminology around impacts and adaptation continues to evolve. At times, 'resilience' has been used interchangeably with 'adaptive capacity'. Each term refers to an attribute of a system that relates to its ability to deal with external stressors, and both can be either constrained or enhanced by internal and external factors. However, as the definition of resilience implies an inherent characteristic of systems to remain at their current state and to provide the same function and structure (Walker et al., 2004), it does not necessarily align well with the goals of adaptation, where change is viewed as a necessary consequence of changing climate.

FIGURE 3: Adaptation will increase the coping range, making systems more resilient, and therefore less vulnerable, to climate change (adapted from Smit et al., 1999).
Text version
Line graph demonstrating our past resilience, potential vulnerability and resilience after adaptation to the climate. The graph exhibits a critical threshold for the climate, when it was stationary, in the past, and how it presently measures up to the threshold. Long term planning and the implementation of adaptation are also demonstrated in the graph, and we can perceive the effect of resilience that could be gained as a result of adaptation in the context of climate exceeding the threshold of the graph.
The definition of 'resilience' introduces two related concepts that are important for adaptation: 'coping ranges' and 'thresholds'. 'Coping range' refers to the variation in climate that a system can absorb without incurring significant impacts. Adaptation actions will adjust the coping range, and similarly affect resilience (Figure 3). A 'threshold' is the point at which significant impacts are incurred (i.e. the coping range is exceeded) or the system undergoes a change of state (i.e. resilience is overwhelmed). Defining thresholds within natural systems is a key objective of many climate change impact studies (International Scientific Steering Committee, 2005), while understanding thresholds in human systems can be key to guiding adaptation decisions. Walker and Meyers (2004), however, have questioned whether thresholds can be defined before they are crossed, and found no examples in the published literature of thresholds being predicted before occurrence.
2.5 TECHNOLOGY FOR ADAPTATION
Technology is frequently cited as a vital solution for the challenges presented by climate change. This is particularly true for mitigation, where a range of innovative new and developing technologies hold promise for providing alternative sources of energy, enabling sequestration of greenhouse gases and enhancing energy efficiency. Technology will also play a role in adaptation (United Kingdom Climate Impacts Programme, 2005). Access to, and use of, technology is commonly cited as a determinant of adaptive capacity. For example, use of water conservation technologies may improve capacity to address climate change impacts on water supply (see Chapter 7). The goals of technologies for adaptation include improved resilience and flexibility, prevention of additional damage and reduction of costs.
Although relatively little research has focused on the actual role of technology in climate change adaptation, the concept of 'technologies for adaptation' is explored in a comprehensive manner by Klein et al. (2006). The term itself (as opposed to 'adaptation technologies') indicates that many of the technologies that may be implemented for climate change adaptation represent existing technologies developed to address issues not directly related to climate change. While the focus in mitigation has been on the development of new technologies, greater emphasis in adaptation will likely be placed on the transfer of existing technologies that are then customized to meet local requirements. In the climate change literature, technology tends to be given a very broad definition, such as "a piece of equipment, technique, practical knowledge or skills for performing a particular task " (Metz et al., 2000), hence encompassing virtually every conceivable adaptation option. A distinction is generally made between hard and soft technologies, the former referring to physical products and the latter to practices and planning. Successful adaptation strategies will generally include both hard and soft technologies (Klein et al., 2006). Further distinctions can be made between traditional, modern, high and future technologies (Klein et al., 2006). In this assessment, the term 'technology' is generally limited to hard technologies.
2.6 SCENARIOS
Scenarios are "a coherent, internally consistent and plausible description of a possible future state of the world" (Parry and Carter, 1998). A scenario is not a prediction, since use of the term 'prediction' or 'forecast' implies that a particular outcome is most likely to occur. Rather, a scenario represents one of any number of possible futures. Both climate and socioeconomic scenarios provide input to analyses of impacts, vulnerability and adaptation measures. They provide a foundation to guide and explore the implications of adaptation and mitigation decisions, and to raise awareness of climate change issues. Scenarios define a range of possible futures that facilitate consideration of the uncertainty relating to different development pathways, with implications for future climate, social, economic and environmental change. For national and regional scenarios extending more than about 30 years into the future, significant attention has been paid to the development of climate scenarios, whereas socioeconomic scenarios remain poorly developed despite the direct linkages between the two.
Climate Scenarios
Most climate scenarios are derived from climate model output, usually from Atmosphere-Ocean General Circulation Models (AOGCMs; see Box 3). Current standard practice in scenario development is to calculate the change between 30-year average AOGCM representations of the future (e.g. 2040 -2069) and baseline (currently 1961-1990) conditions, and to apply these changes to observational data. These changes are generally expressed as simple differences for temperature, and in percentage differences for precipitation. Model output is averaged over thirty years for both the baseline and future time periods to ensure that the longer term climate change trend is captured. The AOGCM-derived changes are referred to as climate change scenarios, or sometimes as change fields. A climate scenario refers to the data that result from applying these change fields to observed climate data, and represents climate information for the future time period (e.g. the 2050s). Owing to uncertainties involved in the projection of future climate (see Box 3), it is important that impacts and adaptation studies consider a range of climate change scenarios. The use of climate scenarios in this assessment is discussed in Section 5.3. Further information concerning scenarios can be found in Intergovernmental Panel on Climate Change Task Group on Scenarios for Climate Impact Assessment (1999).
Socioeconomic Scenarios
Social and economic conditions will not remain static as climate changes, and understanding the likely nature of these socioeconomic changes is important in characterizing vulnerability to climate change. Socioeconomic scenarios, which include information concerning population and human development, economic conditions, land cover and land use, and energy consumption, provide important information for understanding adaptive capacity. Global-scale socioeconomic scenarios extending to 2100 are the foundation of the emissions scenarios in the Special Report on Emissions Scenarios (SRES) of the Intergovernmental Panel on Climate Change (IPCC; see Box 3; Carter et al., 2001). It is unclear, however, whether these scenarios can be meaningfully downscaled for the purpose of impacts and adaptation studies. Socioeconomic forecasts at the national and regional scales may be more relevant for use in impacts and adaptation studies.
BOX 3: Climate modelling
Atmosphere-Ocean General Circulation Models (AOGCMs)Footnote 2
The extreme complexity of the Earth's climate system, involving dynamic interactions between the atmosphere, the oceans, the cryosphere, land surfaces and the biosphere, necessitates the use of sophisticated AOGCMs to project future climate change. These AOGCMs are three-dimensional mathematical representations of the large-scale physical processes of the Earth-atmosphere-ocean-land system, and provide a comprehensive and internally consistent view of future climate change. In AOGCMs, the Earth's climate system is divided into a gridded network of interconnected boxes, and the physical processes that control this system are represented by series of fundamental mathematical equations describing the conservation of momentum, mass and energy. Feedback effects in the climate system, such as those between snow and ice and the reflectivity of the Earth's surface (albedo), are included in the models, although some of these processes are incompletely specified and poorly quantified.
To project future climate, AOGCMs must be provided with information about future atmospheric composition. Future levels of greenhouse gas and aerosol emissions are dependent on a range of factors, including population growth, economic activity and use of energy and technology, so there is a wide range of possible emissions futures, referred to as emissions scenarios. For its Third Assessment Report, the Intergovernmental Panel on Climate Change commissioned a Special Report on Emissions Scenarios (SRES), which describes about forty different emissions scenarios (Carter et al., 2001). Six of the SRES scenarios have been identified as 'marker scenarios' and are recommended for use by the climate modelling community, namely A1FI, A2, A1B, B2, A1T and B1 (presented in order of descending radiative forcing by 2100). At the extremes, the A1FI storyline describes a fossil-fuel-intensive world with very rapid economic growth, global population that peaks around 2050 and rapid introduction of new technologies. The B1 storyline describes a convergent world in which population also peaks about 2050, but with rapid economic changes towards a service and information economy and the introduction of clean and resource-efficient technologies (Carter et al., 2001). Best estimates and likely ranges of globally averaged temperature changes and sea-level rise for each of these marker scenarios are shown in Table 3.
Uncertainty in projections of future climate increases with time. Emission scenarios represent one source of uncertainty related to future development pathways. Although this uncertainty cannot be avoided, it is noteworthy that emission scenarios only become an important source of uncertainty after about 2030 (Intergovernmental Panel on Climate Change, 2007a). A second source of uncertainty relates to differences between AOGCMs in the way physical processes and feedbacks are simulated. These differences result in the different AOGCMs simulating different global warming values per unit change of radiative forcing. New methods for dealing with this uncertainty have emerged since 2001 (Solomon et al., 2007).
Regional Climate Models (RCMs)
Regional Climate Models provide higher spatial resolution (i.e. more detailed) data than AOGCMs by nesting a high-resolution RCM within a lower resolution AOGCM. This means that RCMs are susceptible to any systematic errors present in the AOGCM used (Canadian Institute for Climate Studies, 2002). An advantage of RCMs is their ability to provide information that is more spatially detailed, and hence at a more appropriate scale for climate impact studies (Laprise et al., 1998). At present, however, RCM data are only available for a limited combination of AOGCMs and emission scenarios, and generally do not encompass a full range of plausible futures. Nevertheless, work in this field is evolving rapidly, with analysis and quantification of the confidence and uncertainty associated with RCMs a major area of research (cf. Caya, 2004; Déqué et al., 2005, Plummer et al., 2006).
Case | Temperature Change (°C at 2090-2099 relative to 1980-1999)a |
Sea Level Riseb Model-based range excluding future rapid dynamical changes in ice flow |
|
---|---|---|---|
Best Estimate | Likely range | ||
Constant Year 2000 concentrationsc | 0.6 | 0.3 - 0.9 | NA |
B1 scenario | 1.8 | 1.1 - 2.9 | 0.18 - 0.38 |
A1T scenario | 2.4 | 1.4 - 3.8 | 0.20 - 0.45 |
B2 scenario | 2.4 | 1.4 - 3.8 | 0.20 - 0.43 |
A1B scenario | 2.8 | 1.7 - 4.4 | 0.21 - 0.48 |
A2 scenario | 3.4 | 2.0 - 5.4 | 0.23 - 0.51 |
A1Fl scenario | 4.0 | 2.4 - 6.4 | 0.26 - 0.59 |
aThese estimates are assessed from a hierarchy of models that encompass a simple climate model, several Earth Models of Intermediate Complexity (EMICs), and a large number of Atmosphere-Ocean Global Circulation Models (AOGCMs). | |||
bSea-level-rise estimates are based on observed flow rates from Greenland and Antarctica for 1993 2003. These rates may increase or decrease in the future. If they were to increase linearly with global mean temperature rise, the upper ranges shown in the table would increase by 0.10.2 m | |||
cYear 2000 constant composition is derived from AOGCMs only. |
In Canada, researchers have access to RCM data from the Canadian Regional Climate Model (CRCM) through the Canadian Centre for Climate Modelling and Analysis (CCCma; see http://www.ec.gc.ca/ccmac-cccma/default.asp?lang=En&n=4A642EDE-1); refer to Laprise et al. (2003) and Plummer et al. (2006) for discussions of model sensitivity and validation. The Ouranos Consortium provides support for the development of the CRCM and has utilized scenarios based on RCMs for analysis of climate change impacts (see Chapter 5).
Page details
- Date modified: